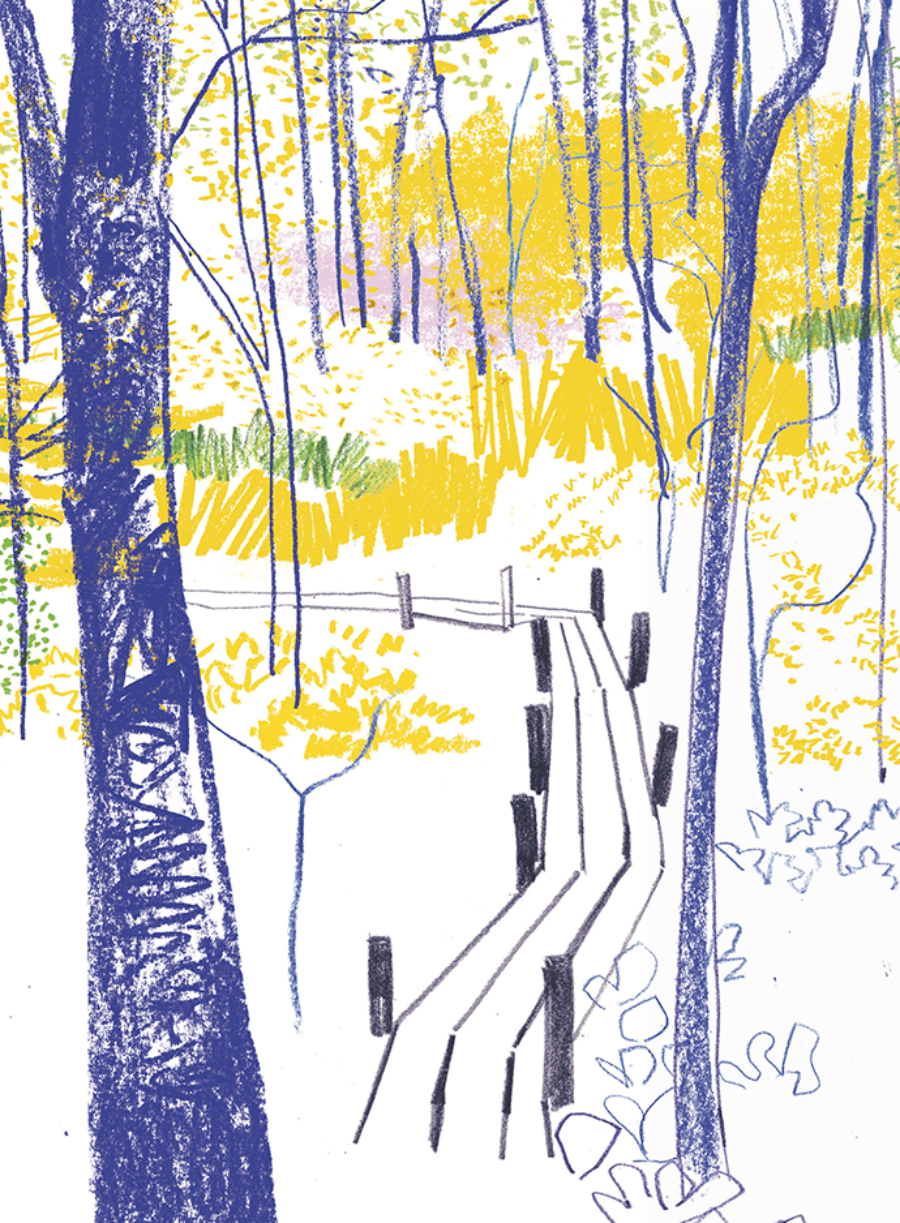
Illustrations by Lara Harwood
Ninety minutes west of Boston, up the road from a Benedictine monastery, lies what is perhaps the most studied forest on Earth. Since 1907, when the first of these 3,850 acres of black oak and red maple was donated to Harvard University, researchers have been designing experiments to better understand the woodland ecosystem—including the longest-running climate-change simulation ever conducted. Around dawn one morning in October, I arrived at a redbrick laboratory at the edge of the forest to meet Clarisse Hart, a graduate student in the forestry program at the University of Massachusetts Amherst who has been the director of outreach and education here for over a decade. Hart’s short brown hair was pulled back in a ponytail and she wore a purple sweatshirt tied around her waist. She spoke with the gentle, pragmatic voice of someone who spends a lot of time around trees. Together we stepped into the autumn air and walked along a wide dirt road into the woods.
As we hiked, I began to notice scientific instruments hiding among the blueberries and starflowers on the forest floor. White PVC pipes emerged from the soil at forty-five-degree angles, shielding cameras that use subterranean flash photography to capture images of slowly growing roots. I spotted a couple of bright blue circles on the ground, each about the size of a quarter, which Hart explained were ant traps, designed to take a census of roving insect armies. Not all the equipment was sophisticated: researchers had stationed laundry baskets at regular intervals to catch falling leaves, which they then weighed to help measure the flow of carbon from the trees to the soil. Hart pointed out something to our right that looked like a partially buried Instant Pot, its lid open and attached by a hinge. Inside I saw nothing but dirt. Without warning, the lid snapped shut, sealing off a small part of forest floor. Hart said that researchers use these devices to measure how much carbon is being released by the soil.
As forests go, Harvard Forest is quite young—an ecological teenager. Each year it takes deeper breaths, absorbing more and more carbon dioxide. Two hundred years ago, a family of farmers used the land as pasture for cows and sheep, but the rise of industrial farming and the opening of the Erie Canal put them out of business. The family sold the farm and used the capital to start a bank. Without constant labor keeping the wilderness at bay, forest crept back over the land. White pine and maple trees gradually filled in the old grazing pastures. “Forests are very pragmatic,” Hart told me. “After enormous changes, they still ask, ‘Where’s the light and water?’ ”
After walking for an hour, through hemlock groves and a bog, Hart and I reached our destination: a thirty-six-square-meter patch of forest demarcated by orange tape. Nothing distinguished the enclosed land from the rest of the forest except a downed birch that had fallen across the plot. In the distance, I could see many more plots that had been delimited in a similar way. In 1991, Hart told me, a team of ecologists had come here to bury heating coils made from the same metal that glows red in a toaster. Their plan was to raise the temperature of these squares of forest by five degrees Celsius in order to figure out how the soil would respond to climate change, anticipating that the relationships between bacteria, fungi, and the trees would shift as the ground warmed up. The experiment, which is now entering its twenty-ninth year, has shown that the way forests react to heat is more complicated than anyone imagined.
We do not understand forests, not really, because we do not understand dirt. Scientists once thought that dirt was simple stuff, responding to temperature fluctuations as mechanically as the mercury in a thermometer. But a forest is an interconnected system: trees rise out of an underground network of roots and microbes that are themselves alive and evolving.
Natural processes in the soil contribute more than six times as much carbon dioxide to the atmosphere each year than does the burning of fossil fuels. Microbes in the dirt release the gas as they consume dead plants and animals. Before the Industrial Revolution, those emissions were offset by the uptake of carbon by plants, but as global ecosystems react to a sudden shot of carbon dioxide, the balance has been disturbed. Even a slight change in how soils behave would have drastic impacts on the environment. “There are something like two hundred billion microbes in a handful of soil, and when you scale their activities up to the planet, it has real implications,” one researcher told me. When something is this complex and obscure, the best approach is to watch and wait. The scientists at Harvard Forest are working on a patch of the future, patiently studying a world they hope will never come.
Jerry Melillo, the researcher who designed the soil warming experiment, knew from the beginning of his career that climate change would be the defining issue of the twenty-first century. After he finished his PhD in forest ecology and biogeochemistry at Yale, in 1977, Melillo was hired at the Marine Biological Laboratory in Woods Hole, Massachusetts, by George Woodwell, one of the first people to raise the alarm about global warming. At the time, anxiety about climate change was already palpable among scientists. “We knew that humans were in a major way disrupting the carbon cycle,” Melillo said, referring to the exchange of carbon among soils, plants, oceans, and the atmosphere. “We knew enough about the biogeochemistry of the planet to know that this was not a good deal.”
Melillo wanted to do research that reckoned with the effects of severe climate change, even if they would not be seen for generations. “Pushing the system to see what happened was the first thing that we wanted to do,” he told me. Melillo and a handful of other scientists around New England joined forces to study how forests recover from disasters, whether natural or man-made, slow or sudden. In 1988, members of the group submitted a grant proposal to the National Science Foundation to turn Harvard Forest into a Long-Term Ecological Research site—a special designation that allows scientists to work on a project for longer than is typically accommodated by the standard three-to-five-year grants. They wanted to compare the shifts brought about by greenhouse gases with the changes the forest had seen before.
The soil warming experiment was a compromise. At first Melillo had talked about building a dome over the whole forest to regulate its temperature, but that idea was quickly deemed too risky and expensive. He had heard about a team of researchers in the Rocky Mountains who had hung infrared heaters from a wire suspended over the landscape, but that study took place in an alpine meadow—trees are simply too tall to cover. Melillo eventually settled on the idea of warming the soil, because it was the portion of the forest ecosystem that was easiest to heat and least understood.
Melillo was inspired by the Denver Broncos, who had buried heating cables under their practice fields to prevent them from freezing in the winter, but it was an entirely different challenge to keep the ground at a stable temperature at a site miles from a city, with only a single power cable connecting the heating source to the electric grid. After months of prototypes and design meetings, a team of young research assistants traveled to Harvard Forest and hiked out to the test site. They carefully unspooled nylon string along the path where the heating cable would go, then dug a trench ten centimeters deep, doing as little damage as they could. They placed the cable at the bottom of the trench and used their heels to push the earth back into place.
Soil changes when it is disturbed. Normally plants release nutrients from their roots—sugars, amino acids, vitamins, and other compounds—which nourish microbes beneath the surface; in return, these microbes provide nutrients to the plants. When a shovel breaks the earth, it pierces through delicate root systems and causes plants to release more food than they otherwise would, throwing the system off balance. Melillo planned for this—he had an assistant dig control plots filled with heating cables, never to be turned on, to understand how the soil would recover from the disturbance. Even without any extra heat, they found that the ground took months to return to its original state.
After the cables were buried, researchers built a shed to hold electrical equipment, including the brains of the experiment: a set of specialized computers that would continuously record data from the soil, turning the heating cables on and off to keep the temperature constant. In July 1991, Melillo turned on the heat and started recording data. Every month since—except in the winter, when the forest is less active and blanketed in snow—a scientist has visited the plot to measure the gases that escape from the soil.
Microbes flourish when they are warmer and have more freedom to move around, so Melillo and his colleagues expected the microbes in the heated soil to consume more nutrients and emit more carbon dioxide as a byproduct. They were right. “The initial response was, in some ways, exactly what you would expect,” said David Foster, a silver-haired ecologist who has been the director of Harvard Forest since 1990. “If we heat up soils, we’re going to release a ton of carbon dioxide. And that’s going to have this very strong positive feedback.” Soils, the team concluded, would exacerbate global warming.
It took ten years for the story to change. In 2001, scientists noticed that the soil in the warmed plots had stopped releasing excess carbon dioxide—its greenhouse gas emissions looked just like those of the control plots. When the scientists looked more closely, they found that the microbes, which had been eating quickly in the warmer environment, were now dying off in large numbers because they were out of balance with the rest of the ecosystem. Eventually the pantry emptied, and the microbes starved. This was good news for the climate: rather than wreaking unending havoc on the atmosphere, the soils seemed to have a natural cap on the amount of carbon they could blow off. This, too, had been a hunch of Melillo’s. “We were thinking there might be a biochemical break built into systems that would eventually stop or at least dramatically slow the feedback,” Melillo said.
Feedback refers to the way the earth responds to warming, either by worsening or mitigating it. Perhaps the most important feedback happens at the poles: when Arctic ice melts, it exposes the dark sea underneath, which sucks up sunlight like hot asphalt, dramatically speeding global warming. On the other hand, trees can sometimes grow faster when the amount of carbon dioxide in the air increases, adding rings to their trunks and absorbing greenhouse gases in the process. International teams of climate scientists have attempted to game out positive and negative feedback to estimate future climate change, and they universally agree that, overall, the earth acts to amplify global warming—the heating from melting ice far surpasses the cooling from supercharged trees. Every bit of carbon dioxide we emit matters that much more because the planet is not looking out for itself. But, the scientists thought, at least soils in temperate forests were off the list of things to worry about. In 2002, Melillo and his colleagues published their results in Science. The experiment seemed complete.
But Melillo decided to continue. He kept the power on and the data coming, which was no small task. Someone had to go out every month to sample the soil, and someone had to pay the power bill for a giant toaster that was always on. “There’s a tremendous difference between running an experiment for a couple of years and running an experiment for thirty years,” said Frank Bowles, Melillo’s engineering deputy. Bowles and his team wrote a detailed manual to ensure that any component could be easily replaced—the idea was that if anything happened to any of the original scientists, or any of the original equipment, the experiment could go on. This planning proved wise: in 2005, the shed housing the computer equipment burned to the ground after a lightning strike, leaving a pile of ashes where the control system used to be. Melillo rebuilt and kept going. He wanted to make sure that there wouldn’t be any surprises—climate change is a long-term phenomenon, after all.
Forests are endlessly inventive, constantly adjusting to dramatic changes. After the glaciers that covered New England retreated, around fourteen thousand years ago, the forests here consisted mostly of spruce and jack pine, species that now thrive much farther north. Over the next six thousand years, white pines advanced onto the landscape, followed by hemlocks, oaks, and beeches as the climate warmed and rainfall increased. Hurricanes swept through every century or so, blowing many trees to the ground. Native people reengineered the forests around them, burning land to control underbrush and clear acreage for farming. Blackberries and raspberries thrived in the charred forests, rapidly regenerating from their surviving roots and taking over the open, sunny landscape faster than the trees could.
Over the centuries, species came and went, but the forest remained, knitted together by an ecosystem of microbes beneath the surface. Thin filaments of fungi spread through the soil in a network that ecologists call the Wood Wide Web, through which trees send nutrients and messages to one another underground. The soil itself is an engine of change. “An equilibrium forest is not really something that happens,” said Audrey Barker Plotkin, a senior scientist at Harvard Forest. “The role of change and disturbance is really fundamental.”
Even so, the disturbances these lands have seen since colonization are of an entirely different order of magnitude. European colonists deforested about half of southern New England in the eighteenth century. Today, only a few thousand acres of old-growth forest remain in Massachusetts. Henry David Thoreau wrote of meadowlarks and bobolinks, songbirds that thrive in grassy environments, but those species were interlopers; after American agriculture moved to the Midwest in the late nineteenth century, forests returned to New England, and the meadow birds began to disappear. “All the landscape is in motion,” Foster told me, “in ongoing recovery.”
By 2008, the soils in the warming experiment had also gone through a kind of recovery. Melillo noticed something strange in his plots: the soil had started emitting carbon dioxide again. Even though the underground environment was as hostile as ever—the nutrient-rich compounds were gone—the microbes were wilier than expected. The natural cap on emissions that the scientists thought they had discovered in 2002 turned out to be temporary. Each heated plot was now emitting 3.6 kilograms of carbon dioxide more per year than were the control plots, a difference only slightly smaller than in the experiment’s early days. When Melillo extrapolated the results, he calculated that over the course of the twenty-first century, warmer soils around the globe will emit the same amount of carbon dioxide as all the fossil fuels burned by humans in the past two decades.
On a cold, bright day in November, I visited the laboratory of Kristen DeAngelis, a microbiology professor at the University of Massachusetts Amherst who has spent years trying to understand what’s happening in these warmer soils. Sunlight streamed into the lab through floor-to-ceiling windows, illuminating fume hoods and solvent bottles. A scientist in a white coat, carefully pipetting microbe samples, stood next to a row of lab benches where dozens of plastic bowls sat heaped with dirt. Each mound was a slightly different shade of brown, like swatches in a Sherwin-Williams catalogue.
DeAngelis, a lively woman with a mop of black curls, joined the soil warming experiment in 2011. She uses genome sequencing to understand how bacteria interact with plant matter and other microbes in the soil. She told me that soils have several ways of adapting to changing conditions. For example, the composition of microbial communities can shift, with organisms that thrive in the new environment coming to dominate the soil. That’s what happened in 2001, when carbon emissions from the plots stabilized: a die-off in fungi made the neighborhood much quieter, and species that required less nutrient-rich soil took over. The new microbial community consumed less food and emitted less carbon than before. In 2008, Melillo and his colleagues thought something similar was happening again, but when they took a closer look at the warmed soil, they found that the species of microbes had not changed much. Something else had to explain the new rise in carbon emissions.
From her experience as a microbiologist, DeAngelis knew that microbes often gain new abilities under stress. She found that the microbes in the warmed plots were better at digesting cellulose, hemicellulose, and lignin—tough, mealy molecules known as “recalcitrant carbon”—than the same species in the control plots. Soils around the world are full of recalcitrant carbon, but scientists had not anticipated that microbes would be able to consume it. The fact that warmed microbes were willing and able to chow down on these molecules was bad news for the climate. For one thing, recalcitrant carbon takes much more energy to eat, meaning microbes emit extra carbon dioxide with every bite.
I followed DeAngelis into what she calls the “dirty room,” where she and her team prepare soil samples for further experiments. Often they try to isolate a single species of bacteria in a petri dish and coax it to reproduce. (To the untrained eye, it looks like making mud pies.) So far the lab has isolated more than six hundred species of bacteria from dirt samples, which they store in a freezer kept at negative-eighty degrees Celsius. Soils behave differently depending on their microbe populations, and it seems that the presence of only a few members of a key species can change the behavior of an entire microscopic ecosystem, like a handful of enthusiastic dancers who liven up a fading party. Given this complexity, biologists need to focus on more than just the most common species if they hope to understand the forest microbiome. DeAngelis and her staff are working hard to collect more bacteria samples—so far they have catalogued only a fraction of the biodiversity that exists in a single gram of soil.
On one side of the dirty room were dozens of test tubes filled with a white, sandy material. These were artificial soils that DeAngelis had created in order to isolate and control the microbial inhabitants, learning how different species behave and interact. “Soil is a living superorganism,” she told me. “If you feed it the right things, like compost or plant root exudates, it grows. It’s extremely slow, but it can grow.” Soils take centuries to form. Over time, minerals in rocks become food for microbes, and then dead microbes become food for plants. Animals play an important role, too. In New England, soils were built by worker ants, which moved oxygen, water, and other nutrients through the dirt as they hauled bits of sediment to construct their homes, improving the soil’s ability to support plant life. (Earthworms, which build soils in many places, are not native to much of the northeastern United States and eastern Canada.)
DeAngelis’s main collaborator on the soil warming experiment is Serita Frey, a professor at the University of New Hampshire who specializes in fungi. Like DeAngelis, Frey keeps a library of samples extracted from the soil, only she stores them in a room kept at a warm twenty-five degrees Celsius. Frey recalled spending hours as an undergraduate sitting at a microscope in a dark room, counting microbes. “I would go out at night and when I looked up at the sky, instead of stars I would see bacteria,” she told me. Together, DeAngelis and Frey are poised to make sense of the forest’s complex subterranean ecosystem: the way that bacteria and fungi work together to decompose organic matter and feed it back to the trees. But even with all the progress that microbiologists have made in recent decades, DeAngelis emphasized that we still understand very little about dirt. “This stuff is literally everywhere,” she said, “and we don’t know how it works.”
Walking through Harvard Forest on that day in October, I wondered what the land might look like after another hundred years. I put my hand against the rough bark of a giant hemlock tree, whose thick limbs spread out from the trunk in all directions. For someone like me, who gets attached to a single tree or patch of woods, it is hard to remember that this forest, in the scheme of things, is lucky. Warmer, wetter conditions in New England will help the forest continue to thrive even as climate change spreads diseases that kill off individual species.
Hemlocks like this one are dying in large numbers again, as they did 5,500 years ago. Now they are being eaten alive by an invasive insect—the hemlock woolly adelgid—that was drawn to these woods as the temperature rose. But the oaks and maples will move in when the hemlocks are eaten away, as the hemlocks moved in when the chestnuts disappeared a century ago. “The rate of forest growth is increasing,” said Jonathan Thompson, a senior ecologist at the forest. “That’s sort of incongruous with what we know about climate change as a whole, which is that it is going to wreak chaos on the world. It is truly a crisis. It just doesn’t happen to be a crisis for that oak tree over there.”
As the forest warms, the carbon dioxide its soils emit will travel far across the globe. Scientists use data from sites like Harvard Forest to write massive supercomputer programs called Earth systems models, which combine everything we know about the atmosphere, plants, oceans, soils, and humans into a simulation. These predictions are never perfect, built as they are on evolving science and assumptions about human behavior, but models have so far aligned with the warming we have observed. “Some people say that if there’s some uncertainty, then we can’t act,” said Melillo. “That’s ridiculous. Every day in our daily lives we make decisions under uncertainty.” We know enough about climate change to know that what is coming will be far worse than what we have seen in recent years.
Since the experiment began, Massachusetts has warmed by nearly one degree Celsius. If the burning of fossil fuels continues at its current pace, the whole Harvard Forest may reach the temperature of Melillo’s plots by the end of the century. But New England may be unusually resilient, as trees here are used to dramatic seasonal swings in temperature. Farther south, tropical forests see fairly stable weather year-round, and the microbes in those soils may not be as quick to adapt to a warmer world. Recent research suggests that the Amazon rainforest will soon transform from a carbon sink into a carbon source, because of warming and other human-driven change. Farther north, the permafrost is melting. The Arctic tundra contains gobs of easy-to-digest carbon that has been frozen for centuries; once it melts, the microbes there could start a feeding frenzy. Scientists expect that the carbon emitted by thawing land up north will be far worse than anything Melillo observed in his warming experiment.
It makes for a strange kind of grief—mourning change in a place that has always changed. The forest seems to ask for stoicism, for us to accept that the hemlocks have left us before and they may leave us again. But fossil fuel emissions have accelerated this transformation, forcing us to bear witness to changes that once were too slow for humans to see, and prompting others that might never have occurred. Perhaps the grief I feel is the sensation of time passing too quickly, the feeling that we are losing things that should have lasted longer. As the soil beneath my feet warms, catching up to Melillo’s experiment, stoicism is no longer an option.