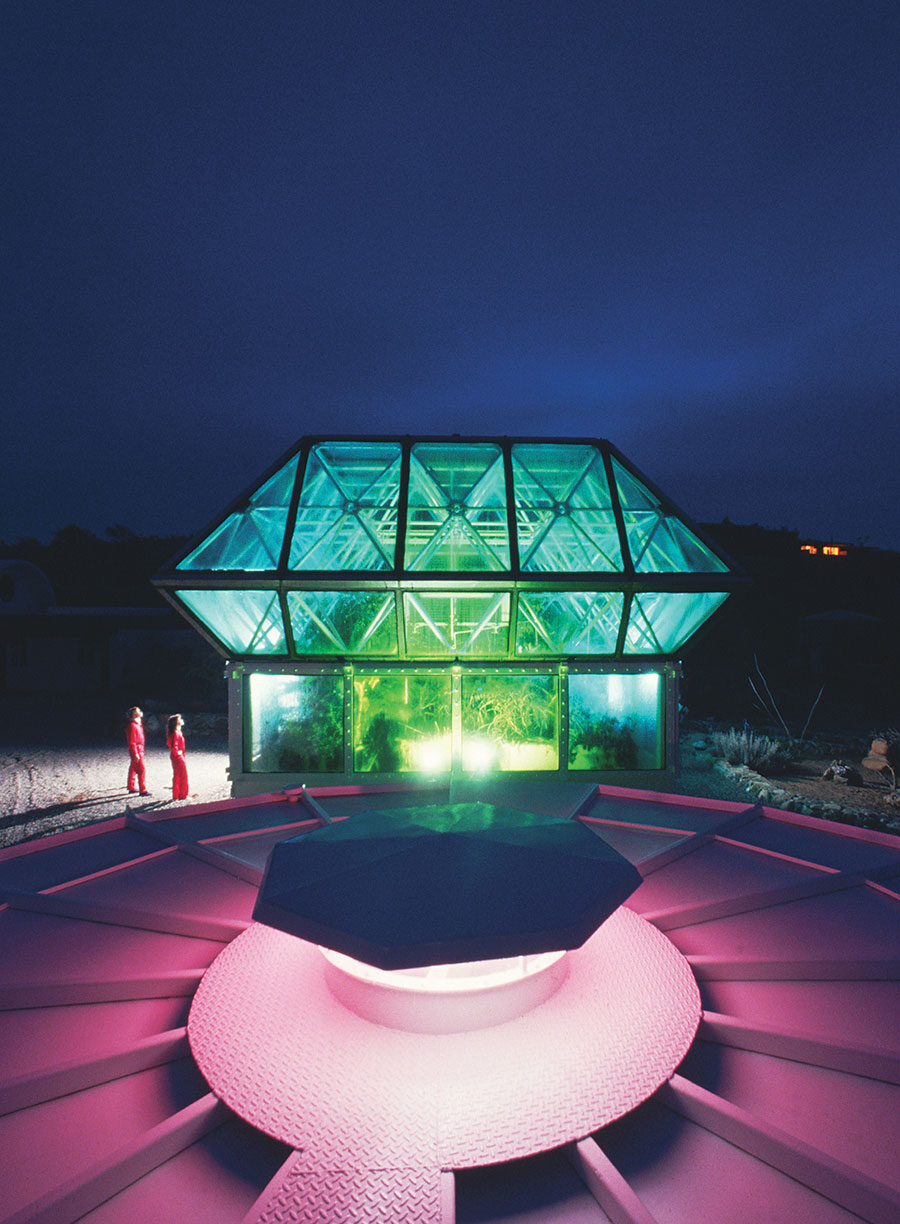
The Biosphere 2 test module, 1988 © Roger Ressmeyer/Corbis/Getty Images
After the bang, much later, after our sun took shape and the earth began to emerge from a molten conflagration that hummed and spat and heaved forth gases that would envelop the entire planet; after bacteria in its waters exhaled enough oxygen to encourage inchoate life, an infinitesimally small portion of which would endure billions of years of evolution to plant seeds and build cities and poison the waters from which it came and turn verdant rainforests to toxic dust; and after that life then began to dream of venturing out into the stars, one man stood in a greenhouse near Tucson, Arizona, and thought about how to re-create it all.
The man, an amateur scientist named Kai Staats, went outside and hosed himself down in the 105 degree heat. Clouds were piling up to the east, but Staats was on deadline and couldn’t let the prospect of foul weather upset his experiment. Staats was a space man. Although he didn’t work for a space agency or have a degree in astrophysics, he had joined a number of endeavors that aspired to launch mankind farther into the cosmos. He had volunteered for a high-altitude ballooning experiment led by an archaeology professor who made DIY space suits. He had worked on a prototype space base in Utah, and tried to advise a doomed attempt to build a colony on Mars. In Arizona, he was embarking on his most quixotic project yet: the construction of a sealed human habitat, one in which people could exist in a miniature environment unconnected to the earth’s atmosphere. Perfecting this technology—known as a closed loop—could allow humans to survive far from the planet for months, enabling long-distance space travel and, eventually, the colonization of other celestial bodies. Staats named his project Space Analog for the Moon and Mars. He called it SAM for short.
SAM wasn’t being built from scratch. Instead, Staats was refurbishing an old test module that had been used for a similar project aborted some three decades earlier. Down a dirt road was the site of that experiment: a glass building of serried arches and a stepped pyramid. Biosphere 2, as it was called (Biosphere 1 was Earth), looked like an extraterrestrial cathedral. It consisted of seven biomes—including a rainforest, a marsh, a desert, a farm, and an ocean with a coral reef—stretching across more than three acres enclosed under white domes. In the early Nineties, eight people had lived inside for two years, shut off from the world except for sunlight and electricity. They were the first of fifty planned crews; the mission was intended to last one hundred years. Instead, it came to be dismissed as a failure by much of the scientific establishment after nineteen of its twenty-five vertebrate species went extinct and carbon dioxide levels shot up so high that half of the crew developed sleep apnea. Its financier instigated an armed takeover, and now the site serves as a research center and tourist attraction.
As the clouds darkened on the horizon, Staats scrambled to patch up SAM’s final few seams. In less than twenty-four hours, he was going to shut SAM’s heavy submarine door with a few volunteers inside. The trial was the first step in Staats’s plan to build a tiny, exportable world. It would last only a few hours, but it was not without risk. The carbon scrubber, a long silver box filled with mineral zeolites that adsorb carbon dioxide, could fail, causing gas levels to rise to dizzying heights, and compromising the cognitive processing of those locked inside. Or, if the pressure rose too high, the building could explode.
Dripping wet, Staats grabbed some tools and climbed atop SAM’s pressure controller. “Hey, Trent?” he called. Trent Tresch, an outdoor expedition guide, was taking time off during the pandemic to help refurbish the test module. “We’ve got a big storm coming in here.”
“We were just talking about that,” Tresch replied.
“I’m wondering if we should try to maybe break from our schedule to try and seal that,” Staats said, running his finger along a joint. “Maybe I should try and spray-foam those now.”
“Okay. We could finish siliconing them too.” A goopy white silicone sealant called Dow Corning 795 had filled every crack in Biosphere 2’s white tetrahedral frame back in the Eighties, so Staats had ordered some online for $14 a tube.
“Well,” said Staats. “That may not be a bad idea.”
The human body is particular about what it needs to stay alive. Surviving well above or below the earth’s crust requires an artificial life-support system to manage changes in pressure and temperature. The body must have near-constant access to oxygen and periodic access to food and water, which means it must also manage the consequences of that consumption: excrement, urine, and carbon dioxide. This isn’t much of a problem on brief space flights—the first astronauts who went into orbit wore diapers. But leaving the planet for more than a few months, let alone trying to colonize a new planet, depends on establishing livable conditions in a continuously regenerating way—on re-creating, more or less, the functions of the earth’s ecosystems.
Most scientific progress to date has concerned the recycling of abiotic factors: air and water. On the International Space Station, for instance, chemical molecules are arranged and rearranged in a never-ending atomic dance. Astronauts breathe air similar to Earth’s, composed mostly of nitrogen and oxygen. The nitrogen is shipped to the station in tanks, but much of the oxygen is produced on board through electrolysis—by running an electrical current through water to divide oxygen atoms from hydrogen atoms. The leftover hydrogen is then transferred into a cylinder called a Sabatier reactor, along with carbon dioxide and a nickel catalyst. The reactor produces methane, which is discarded overboard, and water, which is recycled for drinking or reused to create yet more oxygen. Carbon exhalations that aren’t fed into the Sabatier reactor are captured by zeolites, which, when heated, release carbon dioxide into the vacuum of space.*
What scientists have not managed to figure out is how to sustainably generate and dispose of the biotic variables of ecosystems, such as food and waste. Without a way to cultivate plants, for example, the International Space Station relies on food shipments that are delivered every few months at a cost of $2,720 per kilogram. (The cost was roughly $18,500 per kilogram until SpaceX’s reusable rockets lowered expenses.) The weight of the food needed for a long trip without the possibility of resupply—to, say, Mars—is prohibitive. As for excrement, it’s currently suctioned into plastic bags and jettisoned along with other waste onto a cargo ship that incinerates upon reentering Earth’s atmosphere. Its fiery streak is often mistaken for a shooting star.
Theoretically, astronauts could grow their own food and return their own waste to the soil. Integrating plants and other organisms into a life-support system, at the right scale, could also make use of their other contributions to the biogeochemical cycle, replacing the technology that simulates abiotic regeneration with living ecosystems. After all, plants and animals, including humans, are constantly exchanging carbon through photosynthesis and respiration. One’s effluence is the other’s fuel. By the same token, water evaporates, condenses, and returns to the soil, where it’s purified. Nature, in other words, is a life-support system that wastes nothing, that requires no resupply, and that humans already belong to, which is why scientists have long tried to re-create it for long-duration space missions.
But using biological systems for space exploration always poses the risk of losing control. When you’re dealing with plants, soil, or microbes, things happen beyond human command, even in a highly monitored environment. An aphid can lay waste to the tomatoes, or bacteria can infect the carrots. In big, complex ecological systems, one small imbalance won’t destabilize the whole, but in simpler systems, any slight mismatch in power dynamics can allow one organism to gain a foothold and take over. Living systems thrive in their complexity, whereas artificial life-support systems need to be manageable, reliable, and obedient.
Before starting SAM, Staats tried to account for biotic complexity by creating a computer model of a regenerative life-support system. This was how I first heard about him. I was looking into the history of Biosphere 2, and learned that he had gathered data for his model at the original site. In early 2020, I called Staats to ask about his research, and he mentioned that he was going to try to restart a scaled-down version of the experiment, but with the explicit goal of modeling a Martian colony. He sent me a project brief that included plans to relocate the entire test module to the lawn in front of Biosphere 2’s original entrance. It was an ambitious idea. The module would be expanded with shipping containers, and the entire structure would be pressurized and hermetically sealed. A few months later, he invited me to join the crew that would lock itself inside the rehabilitated test module for its first test run. I had never felt the pull of Mars, but I was curious about what our attempts to go to outer space could tell us about living on this planet. I said yes.
Biosphere 2 sits on a sprawling campus at the edge of a town called Oracle. When I arrived at the gift shop last June, Staats was waiting in the shade, a pair of wraparound sunglasses perched on his baseball cap and a T-shirt tucked into his utility pants. The son of a Lutheran pastor who preferred fixer-uppers, Staats could wield a table saw by age ten. He studied industrial design at Arizona State University, then built a software company, Terra Soft Solutions, which developed an open-source operating system called Yellow Dog Linux. In 2008, he sold the company and made a series of documentaries about the Palestinian territories, the South African Astronomical Observatory, and LIGO, the gravitational wave observatory. On the side, he helped to design a playground in Poland and to build the first astronomical observatory in East Africa. Staats can’t remember the last time he had a regular paycheck. When the pandemic started, another documentary project fell through, and he made ends meet by fixing cow fences.
All the while, Staats nurtured a growing interest in space travel. In 2012, he received an invitation to join a crew at the Mars Desert Research Station—one of two analog space missions run since the early Aughts by the Mars Society, a nonprofit. He spent two weeks living with a group of scientists and engineers near Hanksville, Utah, in a model lander-cum-habitat. He also worked with the founder of Mars One, a Dutch company aspiring to build a permanent colony on the red planet. In 2019, after collecting millions of dollars in fundraising, Mars One declared bankruptcy, and was derided as a scam. But its demise didn’t deter Staats so much as confirm his suspicion that the old model of space exploration, dominated by state-run agencies, wasn’t as unassailable as it once was. With enough vision, patience, and money, he thought, just about anyone could contribute in some way to helping the species survive off-planet. Bas Lansdorp, the founder of Mars One, may have been a con artist, but that didn’t mean that Elon Musk, Jeff Bezos, and Richard Branson would all end up in bankruptcy court. The field’s fragmentation was an opportunity, a chance for closet engineers like Staats, with access to YouTube and NASA’s published papers, to build backyard rockets and develop their own space suits.
According to Staats, the human expansionist project is running out of time. “There really is a limited number of resources,” he told me, referring to the fossil fuels currently needed to power rockets. “If we don’t use what resources are left now to become interplanetary, we will have lost the window and never get off the planet.” (“The alternative to that, of course, is the space elevator,” he added, referring to a futuristic engineering proposal that involves fixing a cable from Earth to a satellite at least 22,000 miles away.) This is a concern he feels viscerally; the thought of being stuck on Earth makes his chest tighten. While he doesn’t consider himself a pessimist, Staats is increasingly certain that human civilization is on a path to self-destruction. Space colonization, as he sees it, is our only option.
Staats walked me through the desert brush, past some abandoned buildings to the test module. He showed me an empty shipping container that would eventually become living quarters, as well as a greenhouse, pocked with holes, in which he planned to build a replica of a Martian crater. “We have a lava tube that’s going in that corner,” he said. “So we can go to the top and actually rappel into the lava tube with space suits.” A company that made synthetic rocks for zoos would build the landscape, and there were plans to install a movie stunt harness to replicate low gravity.
The module itself was a small trapezoidal structure made of steel and glass. Behind it was the lung, a giant white disk designed to calibrate pressure with a large metal pan that would rise or fall as changes in temperature caused the air inside to expand or contract. The exterior wall was marked n1987b. When I asked Staats what it meant, he told me he had registered the module as an experimental aircraft with the FAA. The stencil was its tail number.
Inside, a metal frame made of interlocked tetrahedrons rose up about twenty feet. In an attempt to re-create conditions on Mars, which is fifty million miles farther away from the sun than Earth is, Staats had tinted the windows and painted over the roof. The floor sloped down gently to a basin in the center, and in the back, an underground tunnel led to the lung. There was an electrical panel by the door, an air-conditioning unit near the rafters, and a spigot. Other than that, it was empty, save for a shelf that would later hold the carbon scrubber. “One day we walked by this dilapidated building, just overgrown with cactus and rattlesnakes, and when I looked in the lung I nearly puked, it was so nasty,” Staats said, recalling the first time he saw the test module. “I said, what is this amazing building? I could see the potential. It looks like a spaceship.”
On the day of our enclosure, a cavalcade of trucks and vans arrived carrying students from the University of Arizona’s Controlled Environment Agriculture Center. They were going to install racks of lettuce and spinach to evoke the spirit of the original Biosphere 2 experiments. The students unloaded a few metal shelves and began setting up fluorescent lighting. The roots of the plants, entwined in spongy mineral wool soaked in fertilizer, were loaded into trays and long PVC pipes, rigged up with pumps that would continuously circulate water. Staats radioed John Adams, the Biosphere 2 deputy director: the students needed glue. By midafternoon, a few hours behind schedule, hundreds of lettuce and spinach plants tufted haphazardly from the pipes.
After lunch, Tresch appeared with the carbon scrubber and began filling it with zeolites. He had finished assembling the machine on the kitchen table in the apartment he shared with Staats. Adams and Katie Morgan, one of Staats’s colleagues, arrived as well; they would be the other two volunteers with us inside SAM. Morgan had brought a bottle of champagne to celebrate if the module managed to hold its seal. She reminded everyone that no matter what happened, she had to be on a flight to Jacksonville, Florida, in a fewdays because her family was participating in a golf cart decorating competition for the Fourth of July. They would all be dressing as alligators. “If you die, I’ll fly out,” offered Morgan’s friend Britney, another Biosphere 2 employee.
“Let’s walk through procedures and establish some goals,” said Staats. He didn’t think we would have to be enclosed for more than four hours before we would start to see the kind of impact he expected: a rise in carbon dioxide and a decrease in oxygen as we depleted what had been sealed inside. “John, you expressed some concern about the oxygen being too low. What point do you think is too low?”
“I don’t think we want to get below 15, 14.5 percent,” Adams said. Oxygen is only about one fifth of the air we breathe; the rest is mostly nitrogen, with a small amount of argon and faint traces of carbon dioxide, helium, and neon. But once atmospheric oxygen falls below 19.5 percent, human cells begin to stop functioning. Below 14 percent, the brain starts to falter.
“So, our cutoff, our minimum threshold, is 14.5,” Staats said.
“Obviously, if anybody feels short of breath, has headaches, heart rate increases. . . ,” Adams said.
“What if I feel amazing?” Staats asked.
“It’s not all about you, Kai,” Adams said. Everyone laughed.
They agreed to turn the carbon scrubber on once carbon dioxide levels reached 3,000 parts per million (seven times the normal atmospheric level), although it was unclear how much oxygen would be left at that point.
A few moments later, Staats announced that it was time to begin. “Cute little things aside, it’s time for science,” he said. One by one, we stepped into the module, leaving a small crowd of onlookers outside. He checked to make sure everyone had water. “If you have to go to the bathroom, go now!” he said. Tresch had a bag of Doritos, and there was some disagreement over whether they could be eaten inside, because Staats couldn’t stand the smell. Finally, Staats pushed the heavy white hatch closed and tightened the seal with a long metal lever. It was muggy and the air was still. We sat down around a plastic table, and waited.
The first life-support systems were created by NASA in the late Fifties for Project Mercury, a series of suborbital and low Earth orbit flights that lasted up to thirty-five hours each. Mercury’s capsules reached an altitude of nearly 200 miles, some seventeen times higher than the 60,000-foot point at which pressure becomes less than one pound per square inch and water boils at 98 degrees Fahrenheit, the temperature of the human body. Without pressurization, bodily fluids start to bubble and evaporate. To keep the Mercury astronauts alive, their capsules were equipped with eight pounds of pure oxygen and a system that metered it out to maintain a pressure of around 5 psi (atmospheric pressure at sea level is around 15 psi). The carbon dioxide they exhaled—over two pounds per astronaut per day—was funneled through canisters, where it reacted with lithium hydroxide pellets to become lithium carbonate; the canisters were later discarded. If they got hungry or thirsty, the astronauts could eat tubes of pureed beef or drink Tang.
Even in those early days of spaceflight, scientists understood that long-term missions would require the creation of self-sufficient bioregenerative systems. In the Sixties, the USSR developed a 1,300-square-foot test chamber that connected by air duct to a quag of green algae, which consumed carbon dioxide and expelled oxygen. Researchers living in the chamber could eat the algae, but they found it so repugnant that Soviet scientists had to introduce other, more appetizing plants, such as wheat and cucumbers. The program grew to include a facility called Bios-3, an underground structure where cosmonauts lived for months at a time off the oxygen and calories supplied by crops grown under lamps; black-and-white photographs of the facility show tightly planted stalks of wheat, bent over like windswept beach grass. When scientists attempted to grow wheat on the Russian space station Mir in 1996, the lamps broke and the crop failed. The second crop seemed to thrive at first, but when researchers examined the specimens after they returned to Earth, the plants turned out to be sterile.
Attempts by the European Space Agency (ESA) to build a biological life-support system have similarly revolved around algae—namely Arthrospira, a cyanobacterium that looks like fusilli bucati pasta. Arthrospira is an efficient consumer of carbon: one hectare of it can fix over six tons of carbon, whereas a hectare of trees can fix four at best. The algae is also “very digestive,” according to researchers, but given astronauts’ distaste for it, the ESA loop features a food compartment where crops such as beets and lettuce are cultivated. Excrement in the system is liquefied anaerobically by high-temperature bacteria and gradually broken down into ammonium, volatile fatty acids, and, eventually, nitrate, which is used to fertilize the plants. This approach, highly compartmentalized with each chemical transformation contained in a dedicated, separate chamber, allows scientists to isolate the forms of life whose functions are useful to them. But after three decades, researchers have yet to enclose a human inside.
NASA has also dabbled in space agriculture. In the late Nineties, it conducted experiments at the Johnson Space Center in Houston called the Early Human Testing Initiative, enclosing volunteers in sealed chambers for up to three months at a time. In one experiment, the oxygen for a single crew member was supplied by 22,000 wheat plants. A more ambitious project to enclose four people, named BIO-plex, was planned for the early Aughts, but was ultimately shelved because of budget concerns. Still, NASA researchers have continued work on space agriculture, albeit on a more modest scale. A few years ago, astronauts succeeded in growing lettuce aboard the International Space Station in a miniature garden called Veggie.
Most recently, the China National Space Administration has collaborated with Beihang University to build Yuegong-1, or Lunar Palace 1, a sealed structure with small apartments and two growing chambers for plants. Beginning in 2018, eight student volunteers lived in the capsule, rotating in groups of four for over a year. Their diet consisted of crops they grew, including strawberries, along with packets of mealworms fed with biological waste. Like the ESA’s loop, carbon dioxide was cycled through plants, which were enriched with nitrogen from processed urine. Yet even Lunar Palace 1 fell short of being a truly closed system. While it managed to recycle 100 percent of its water and oxygen, it managed to do so for only 80 percent of its food supply.
As space agencies have haltingly investigated biological life-support systems over the decades, they have been joined by an increasing number of enthusiasts operating outside the purview of government research. The largest of these projects, and the only one that set out to establish a completely closed system, was Biophere 2. Behind the project was a commune-living theater troupe accused of being a cult and bankrolled by the scion of an oil tycoon. Their leader, a charismatic beatnik named John Allen, had an MBA from Harvard and kept company with Abbie Hoffman. They were inspired by the work of the American architect Buckminster Fuller, whose theory of synergetics led them to depart from traditional scientific methods that aimed to isolate and understand single variables. Instead, they wanted to initiate multiple processes at once to see what might emerge.
Things began to spiral out of control almost immediately. Once the system was sealed, oxygen levels inside started declining and carbon dioxide levels began to climb. The volunteers tried addressing the problem by cutting down inedible grasses and preventing them from decomposing, and by filling every possible corner of the habitat with new plant life, but to no avail. With the supply of breathable air dwindling, they decided to inject liquid oxygen directly into their atmosphere.
Shortly after the first mission ended, scientists at Columbia University figured out what had happened. Among the 30,000 tons of dirt brought into the enclosure were rich soils meant for the rainforest biome. These soils were teeming with microbes that had similar metabolic processes to humans. If anything, given all that additional animal life, carbon dioxide levels should have increased even more than they did, but calcium hydroxide contained in the enormous concrete foundation reacted to and captured carbon dioxide through a process called carbonatation. In so elaborate a system, it’s nearly impossible to account for everything.
Improvisational, frenetic, and fueled by romantic ambitions of otherworldly life, Staats’s project embodies in many ways the ethos of Biosphere 2. Throughout his renovations of the test module, Staats has spoken with members of the original crew, including Taber MacCallum and Jane Poynter, founders of a company called Space Perspective that aims to take tourists on high-altitude balloon rides to the stratosphere. The couple, who have long dreamed of traveling to Mars, is also slated to be the first to test a long-term enclosure of SAM once it is fully up and running. Staats joked that he would have chocolates waiting for them on their pillows.
After sealing the door, we all settled around a folding table. Staats and Adams started talking about Linda Leigh, who spent three weeks sealed inside the test module by herself as part of the original Biosphere 2 team. “I would rather fly to Mars and be seven months alone in a spacecraft than be with someone else,” Staats said. It struck me as revealing that someone so interested in space exploration should also be so preoccupied with being alone. The stuff from which a closed ecosystem is constructed—the plants that breathe with us, the soils in which we decay, the bacteria and fungi and viruses that live inside and around us—underscores the interdependence that sustains life. So intricate are these relationships that they may never be perfectly rendered in simulation, even in simplified form. I thought about a conversation I had months before with Francesc Godia Casablancas, a chemical engineer who runs the ESA’s pilot plant in Barcelona, one of the most complex biological life-support systems ever developed. He told me that no matter what, his systems would always lose efficiency over time; the simplified biological cycle built by scientists in a series of reactors would never be “a perfect world.” Living on a planet that still harbors secrets seemed to me like the opposite of being alone. There is a strange kind of companionship in the tension of not knowing, in the fact that the systems supporting life on Earth operate beyond our control.
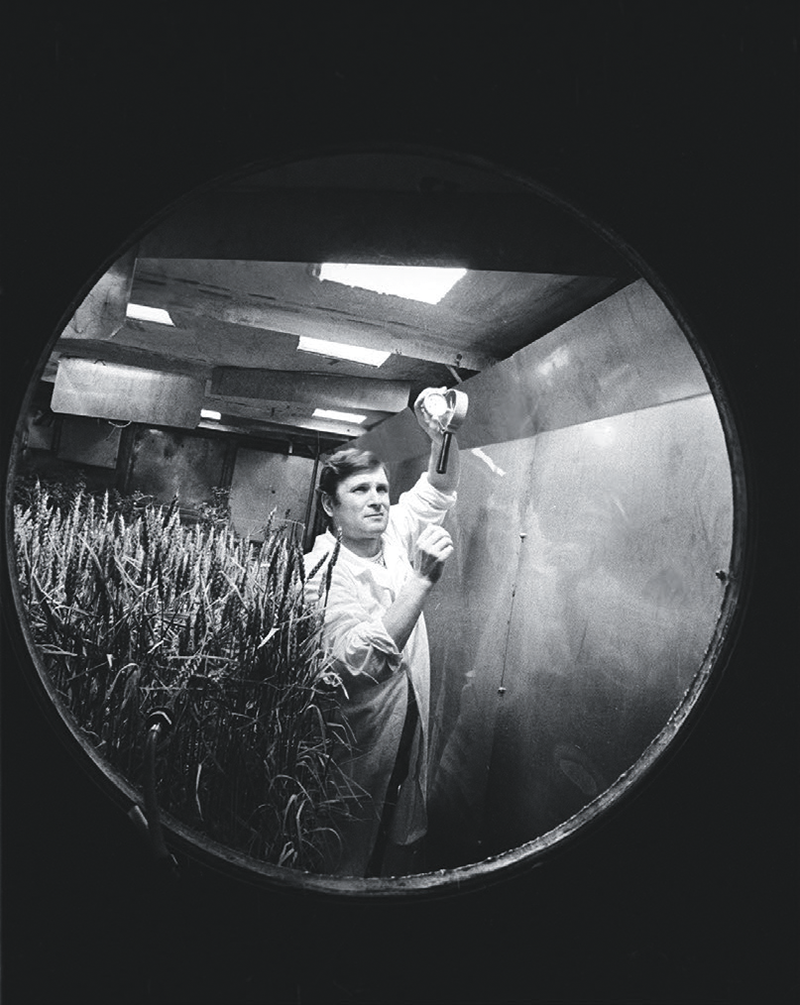
A researcher analyzing changes in airflow at the Bios-3 station © Sputnik/A. Belonogov
Over the next few hours, we tried to play a rocket launch simulation board game called Xtronaut, whose instructions and game play seemed indecipherable. After about forty minutes, Tresch suggested we do an Instagram Live video, for PR’s sake, but Staats didn’t have the app on his phone. He texted his girlfriend, Colleen, who helps manage the project’s social media, to see if she could upload something. We checked the carbon dioxide levels. They were at 953 ppm, only a couple hundred higher than when we started. It was taking longer to increase than Staats expected, and he wondered aloud about how to speed things up. Tresch suggested that we turn the scrubber on at 1,000 instead of 3,000 ppm. Morgan said she was hungry. “We wouldn’t be very good on a long-term space mission,” Tresch said.
Outside, the afternoon sunlight began to soften. A documentary filmmaker friend of Staats’s who was responsible for monitoring the lung reported that it had sunk by a couple of feet since we had started the experiment; the temperature inside had fallen by ten degrees—to 91—but outside it had only fallen by four. Around the two-hour mark, we measured the oxygen concentrations in our bodies. We were fine.
After more than three hours, carbon dioxide levels were slightly higher than 1,500 ppm. Tresch turned on the fans that would pull air through the scrubber’s zeolites at 7,500 cubic feet per minute. Inside the carbon scrubber’s metal encasement, the fans emitted a low hum. Minutes passed, and the sensors showed carbon dioxide levels continuing to rise. Staats began to consider questions they hadn’t answered beforehand. At what density do zeolites function best? Are cylinders the best shape for the job? Was the carbon dioxide moving through the cylinders too quickly? They tinkered with the fans, turning up the speed to see whether it would accelerate the carbon dioxide drawdown. “What’s the CO2 right now?” Adams asked.
“1,561,” said Morgan.
“It’s not going down,” Staats said.
“It’s not going down,” Morgan said.
We waited another hour, nervous and tired. The board game had been long abandoned. We started putting together a robot designed to farm crops, but left it only partly assembled; there weren’t any beds that needed tending anyway. Nobody knew why the carbon dioxide levels had risen so slowly, or why oxygen stayed constant around 17 percent, or why the scrubber didn’t work. We forget how much we don’t know, Staats told me later; it’s not bad to be reminded.
Outside, some graduate students had gathered in the arc of light around the test module to watch us through the glass. I thought again about the eight original volunteers, stuck for two years inside a world of their own making. They subscribed to the Gaia hypothesis, which posits that the planet is a single, self-regulating organism; that the earth’s conditions aren’t just uniquely sweet to human life, but inseparable from it. They described a sense of harmony with the atoms that flowed through them and the surrounding plants. Once we entered the test module, though, that kind of harmony seemed like a distant dream. Instead, our chemistry was doing things we hadn’t anticipated as we played at launching rockets. I wondered whether Staats was right, whether we as a species would be compelled to seek some new terrain, a foreign place where our appetites might grow, expanding and expanding, like the universe itself. It was after eight o’clock; we had been locked inside for four hours. Soon, we would leave the new world, trailing our chemical effusions behind us, and we would go back into the old one, where stars freckled the dark atmosphere and the students waited with lemonade.