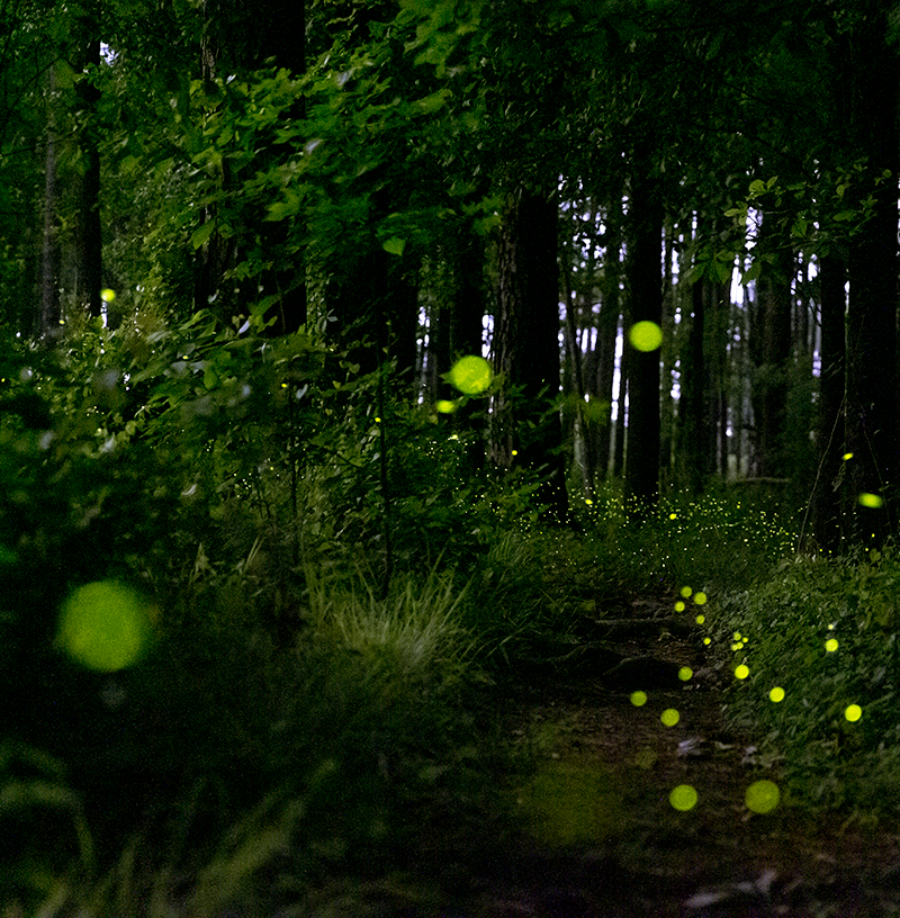
Photuris frontalis illuminate Congaree National Park, South Carolina © Mac Stone
It was a mild May evening in South Carolina’s Congaree National Park, and Raphael Sarfati stood on a trail beneath a loblolly pine he’d chosen as a landmark. The horizon to his left glimmered with light from the nearby city of Columbia, while the tree marked the border of a dark and wild wood. Slanted rays of twilight burnished the trunk, which stretched for perhaps eighty feet before dissolving into an oval of green. The pine, with its fire-blackened base, was probably not yet fifty years old, a juvenile in a bottomlands ecosystem with thousand-year-old bald cypresses. It was the sort of tree Sarfati could recognize in the night, and so it served now as a cue to plunge into the undergrowth toward his field site.
Sarfati is in his early thirties, with curly hair, a compact frame, and a congenial French accent. He moved deftly despite being weighed down by camera bags, tripods, and a bulky poster that resembled a black-and-white checkerboard. Congaree occupies a natural floodplain, and the ground was soft and black and uneven. He stepped high to clear logs lined with turkey tail mushrooms, and to avoid the thick, looping vines that cascaded from the branches above into cushions of dried leaves. When he stopped at a clearing tufted with wispy grass, the woods felt deep and enveloping, though we couldn’t have traveled much more than a hundred yards.
“I try to set the cameras up in the same place every night,” Sarfati said, dropping his gear in a stagelike space framed by two downed trees. What he wanted to capture, what he’d been waiting to see for the past four nights, was the peak activity of Photuris frontalis, a species of firefly that ornaments these woods with rhythmic, synchronous light for a few brief weeks each spring.
Firefly synchrony, in which male fireflies congregate and flash together in an astounding mating ritual, was for many years believed to be rare and largely confined to stable populations of the Southeast Asian genus Pteroptyx. It was only in the Nineties that the Tennessee naturalist Lynn Frierson Faust introduced biologists to years of her meticulous observations, revealing synchrony to be far more widespread among fireflies of the Eastern United States. Ever since, firefly synchrony has become an increasingly well-known and celebrated spectacle, drawing roughly thirteen thousand visitors to Congaree National Park each spring, and a similar number to Great Smoky Mountains National Park, where another species, Photinus carolinus, glows in a similar fashion.
More than two thousand species of fireflies exist, populating every continent except Antarctica. Their adult lives are brief, often lasting just a few weeks, and their flashes are part of what Faust described as a “desperate mating effort.” But in addition to attracting mates, their distinctive flash patterns serve to broadcast distress and even to deceive, as in the case of female Photuris, which imitate the flashes of other species’ females to lure males and devour them. Male Photuris have also been observed to mimic other males, an enigmatic behavior that may represent an attempt to trick the females they know are hunting into mating instead.
Unlike Faust, Sarfati is not a naturalist. He’s not a biologist either. He grew up in Paris, the son of an Algerian-born father and the grandchild of Hungarian émigrés, with an early awareness that he was expected to achieve. He grew fascinated by atoms at around age five, and went on to earn a doctorate in physics at Yale, where he studied soft matter (a topic esoteric enough that Sarfati seemed mildly embarrassed whenever I asked about it). During his first postdoctoral fellowship, at the University of Colorado, he focused on polystyrene beads that measure one thousandth of a millimeter. Then he heard about a professor named Orit Peleg, who used physics, computer science, and chemistry to study bees, and transferred to her lab. Last spring marked Sarfati’s second year doing experimental field work in Congaree as Peleg’s postdoc, having traded his studies of microscopic particles for the realm of living, breathing animals, or what Sarfati likes to call “thinking objects.”
His first task in Congaree was to set up cameras to function as “digital eyes,” which could sense light as well as humans but perceive depth much more capably. Sarfati wore a long-sleeved shirt from an ultramarathon he’d run in the Rockies and a headlamp around his neck like a pendant. He couldn’t complain about the conditions: no yellow flies yet, and only moderate heat. Likewise, he hadn’t heard or seen any of the huge wild pigs that had snuffled at the margins of his site the previous year.
He began fiddling with two cameras, positioning one on a tripod beside an American holly and the other, roughly parallel, next to a pine. Then he walked to the tip of an imaginary triangle in front of the cameras, where he placed the checkerboard atop a collapsible easel. He used the board to calibrate the cameras, then started filming and waited for the gloaming to sink into night.
The first fireflies appeared haltingly: just a few random flashes scattered across the understory, like flames from brittle white sparklers. “This is a frontalis right there,” Sarfati said, pointing at an insect blinking a foot above the ground. “It’s flashing about three times every two seconds, like this kind of snappy flash. And it’s moving more or less in a straight line. Not exactly, but it’s not doing complicated loops or patterns.”
Sarfati spotted a trio of frontalis drifting past low bushes and made finger guns at them, popping his wrists up and down to match their beat. “Look at these,” he said. “One, two, three. Three of them in sync.” I mentioned that I’d thought you needed hundreds, if not thousands, of fireflies to trigger synchronicity. “That’s what a lot of people think, the peer pressure, that you need a lot of common influences,” Sarfati said. “But no, they really want to do it!” Two fireflies, for instance, might be in sync with each other, but not the entire swarm. The famous mass synchronous displays, which tourists in some places must enter lotteries to witness, begin in small, barely discernible groupings. “But if you start to have higher densities, you’re connecting all these dots together, these networks of connection and then ‘Boom!’ ”
The sky had grown dark, and a thundering chorus of crickets or frogs arose where there had previously been trilling wrens. At almost nine o’clock, the woods exploded in rhythm, just as Sarfati had predicted. Countless cold points of light flashed and extinguished with uncanny regularity. The synchronicity was so precise that the fireflies resembled a single luminous organism hovering above the forest floor. “Oh, wow,” Sarfati said softly. “This is good. It’s really good.” Puddles of ethereal moonlight had pooled near Sarfati’s feet, but they seemed suddenly dull and ordinary. Thousands of frontalis glowed and dimmed in every direction, their pulse steady and ancient.
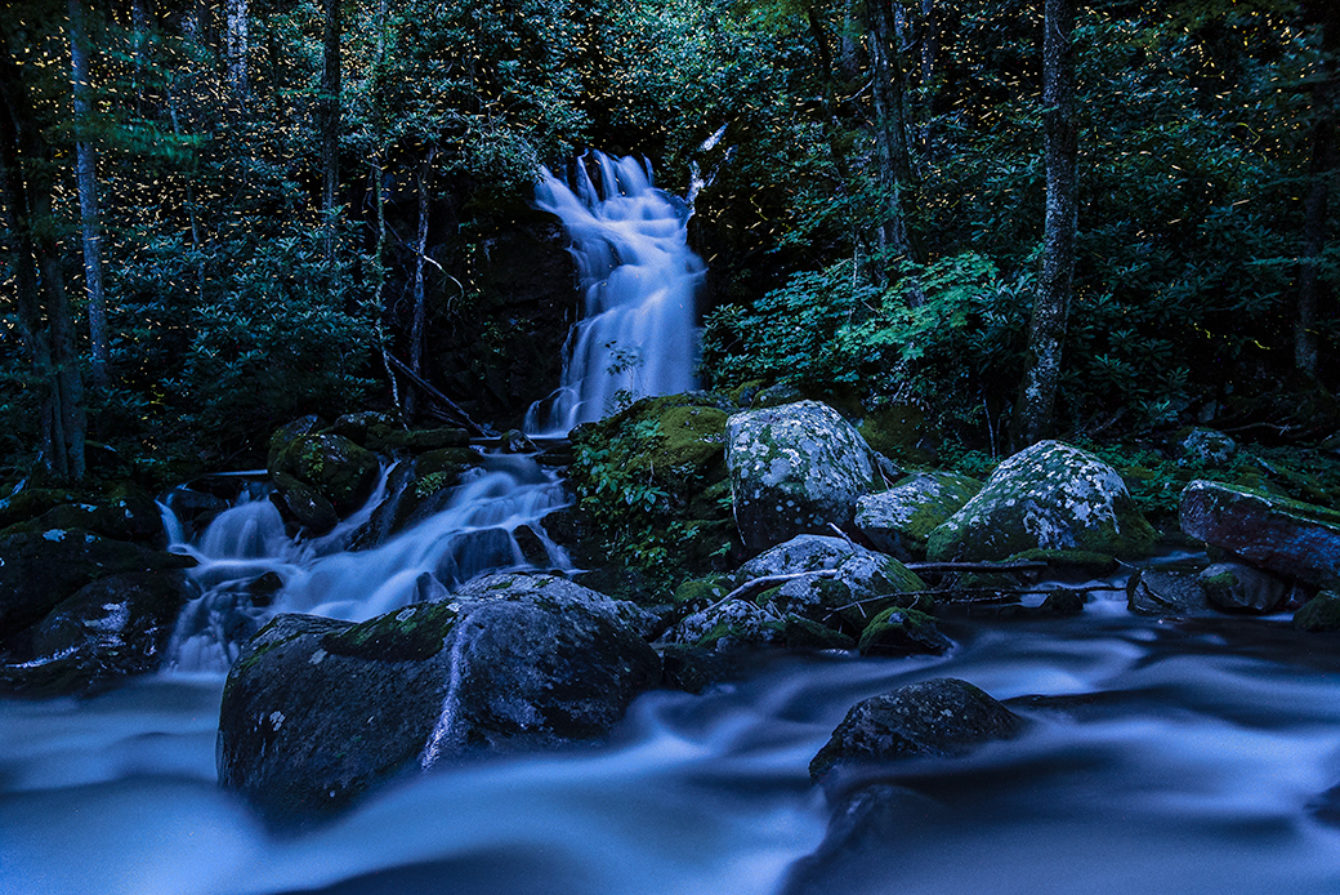
Fireflies along the Big Creek Trail, Great Smoky Mountains National Park, North Carolina © Spencer Black
The firefly pioneers of the twentieth century were Elisabeth and John Buck, a married couple who began methodically studying fireflies and theorizing about synchronicity in the Thirties. As a doctoral student, John outfitted a darkroom to simulate a natural cycle of day and night, filled it with fireflies, and set up a sleeping bag so that he could observe their behavior over twenty-four-hour periods. Later, the couple’s projects would take them to Jamaica, Borneo, Thailand, and Papua New Guinea. Over their long and celebrated career, the pair studied various types of bioluminescence, including those found in marine organisms, but they always returned to the puzzles of fireflies and synchronicity. “Just what capability communal synchrony demands is a question that does not come easily to us as human beings,” the Bucks wrote in 1976, “because we accept our own ability to dance or to march in unison as being second nature.”
Indeed, although Western travelers had been documenting sightings of synchronous fireflies for centuries, researchers who preceded the Bucks were often skeptical or even hostile to the notion that invertebrates with millimeter-size brains could engage in such sophisticated behavior. They dismissed reports of firefly synchrony as accidental, as a byproduct of humidity or tree sap, or as an illusion caused by a twitchy eyelid.
It’s now obvious that firefly synchrony not only exists but has many analogues in nature, where seemingly simple animals organize themselves in staggeringly complex ways. Spiny lobsters form single-file lines on the ocean floor and, like cyclists drafting in a pace line, move faster than any single crustacean might on its own. Vast schools of fish execute intricate high-speed maneuvers—splitting and re-forming behind a predator, for example, or bursting radially—and rarely bump into one another. Giant honeybees nesting on open combs repel predatory wasps by forming spiral waves that spread through the swarm within milliseconds. And most of us are familiar with the sight of starlings wheeling in great dark flocks across the sky, twisting and turning at thirty miles per hour, maintaining a tight choreography even as their numbers swell to the tens of thousands.
How does order arise in the absence of leaders, amid the chaos of thousands of moving, sensing, thinking beings? What, if anything, are these groups trying to achieve? Sir John Bowring, an English governor of Hong Kong, wrote in 1857 that the fireflies’ “light blazes and is extinguished by a common sympathy,” framing synchronous light displays in plainly human terms. A naturalist writing a half century later proposed that the virtuosity of starlings might be explained by avian telepathy.
Such questions might seem like natural territory for biologists, but they were first embraced by chemists and physicists. They wondered whether mathematical models that explained matter could be adapted to different systems. If biologists traditionally looked at animals as organisms within ecosystems, physicists analyzed them as particles in motion that interacted with forces, modeling the behavior of flocks and schools by using the rules that explain magnets, self-propelled particles, and maximum entropy.
Many of these quantitative researchers were inspired by the dawning science of complexity, a field embodied by the interdisciplinary Santa Fe Institute, which opened in 1984. Santa Fe acolytes chased the notion of universal phenomena that share the same general mathematical descriptions. The idea was that dazzling complexity—be it a chemical reaction or termites building a mound—might arise when individuals in a system follow similar sets of rules at the local level.
A shortcoming of this early work was that it was almost entirely computational and theoretical. Researchers who ventured into the real world to conduct experiments struggled with the limits of what their cameras and computers could do. One famous study that involved filming starlings, by the Italian physicists Andrea Cavagna and Irene Giardina, relied on an image-matching algorithm that took two years to build. Other early researchers did statistics by hand: a staggering proposition when faced with thousands of animals.
“In the lab, I have what are relatively inexpensive cameras right now that are imaging at one hundred frames per second,” said Nicholas Ouellette, a professor of civil and environmental engineering at Stanford who studies midge swarms and the flocking behavior of jackdaws. “I can, you know, in a day or two, generate tens of millions of data points that I can do statistics on.”
This data revolution lets researchers test the collective behavior models from previous decades and propose new ones. In many ways, the study of animals using quantitative tools and methods is still in its infancy, wide open for discoveries that augment our understanding of evolution, animal communication, animal sensing, and possibly cognition. Many of these insights will start with fundamental questions about natural phenomena that are at once deeply familiar and stubbornly hard to explain. “What is it about a flock that makes it a flock?” Ouellette asked. “What is it about a swarm that makes it a swarm, and not just a bunch of things flying around?”
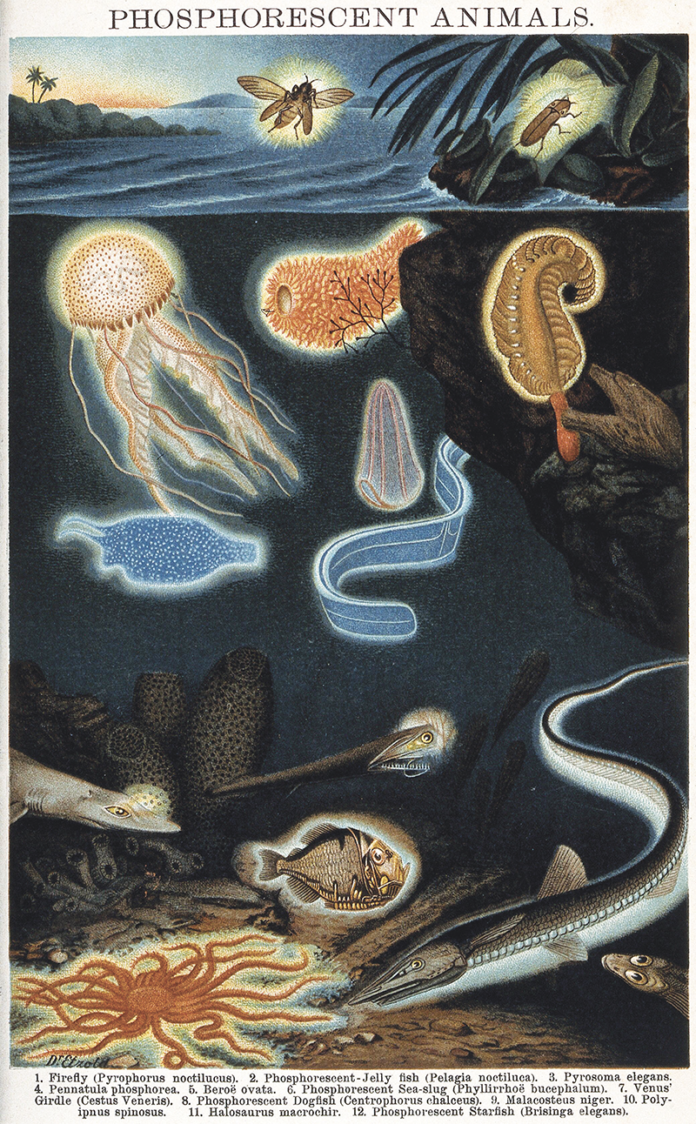
Phosphorescent Animals, after Dr. Etzold. Courtesy Wellcome Collection
One evening in Congaree, I watched Sarfati’s adviser, Peleg, lift a petri dish containing a male firefly that had fallen on its back. It had switched from its normal, unhurried flashing to a more alarmed frequency, its lantern glowing quickly and urgently, a message of distress as plain as any warning beacon of human design. “In the case of fireflies, the communication is a light pattern, kind of like a flashlight that turns on and off like Morse code,” Peleg said.
She was working at a site about a quarter mile from Sarfati, on a gravel track that shone white beneath a nearly full moon. A serene first-year graduate student named Owen Martin had erected two tents. In one, they planned to place individual fireflies to see if they would match their flash frequencies to that of an LED robot; in the other, they planned to introduce fireflies one by one, or a few at a time, to observe how they coordinated the timing of their flashes.
Peleg quizzed Martin on the steps he’d taken to set up the cameras, and his responses underscored the differences between building theoretical models and testing them in the real world. “Checked to see they’re at max ISO,” Martin said. “They’re on fourteen-forty resolution, sixty frames per second, three-hundred-and-sixty video mode, pointing the same direction, calibrated.” It would’ve been easy to leave a lens cap on, or botch the ISO setting, or fail to zip up the tent and let an entire night’s subjects twinkle away into the foliage. Though their equipment was high tech, the experiments echoed some of the classic work done by the Bucks, who once netted scores of synchronous fireflies in Thailand and set them loose in their hotel room to see how they behaved.
Sarfati and Peleg are fairly confident that fireflies synchronize by following visual cues, but why males would flash as a collective when nature is full of males trying to distinguish themselves—consider the elaborate courtship dances of various birds, or the headbutting of rams—is another question. One hypothesis is that fireflies synchronize to reduce visual clutter, making it easier for females to locate them. But Peleg and Martin were interested in how fireflies adjust their flash frequencies, not why. And first, they needed to catch them.
Peleg walked along a raised boardwalk that ran perpendicular to the path where their tents were set up. She carried a butterfly net and made confident sweeps above the vegetation, netting fifteen fireflies in the time it took Martin to snag five. In the nets, the glow of the fireflies appeared green rather than white, like sparks from a strange fire. Although Peleg’s data on frontalis was preliminary, her team had collected intriguing findings while observing Photinus carolinus, the synchronous species from the Great Smoky Mountains. “When we take them out of the swarm, they behave very differently,” Peleg said. “In isolation, they behave one way. And when they’re among their peers, they behave very differently.” While frontalis synchronize in a steady rhythm for hours, carolinus flash in bursts, followed by communal pauses. But a lone carolinus doesn’t follow the same pattern; its flashes and rests are sporadic. Once in the collective, it’ll adjust its pauses to match the group’s rhythm. In other words, the males aren’t preprogrammed to a constant frequency; it’s peer pressure that changes the timing mechanism in their brains.
The field of collective animal behavior is, in essence, a science of social influence. Explaining how one electron influences another is relatively easy, considering particles do not sense or think, or acknowledge rank, or select lifelong mates. Animals, on the other hand, evolved to make decisions within complex social contexts that are often riddled with loyalties and hierarchies. They see, hear, and smell; some detect pheromones, vibrations, or pressure. One animal might influence the decisions of another, but not vice versa, or the actions of animal A may affect those of animal B, which in turn circle back to influence animal A. (Now extend those simple influences between two pairs to populations of hundreds or thousands.) Sometimes animals cooperate when it’s advantageous, but they can also be genetically selfish. They can also cheat, which distances them from the sorts of comprehensively predictable patterns that physicists are trained to search for.
Nevertheless, research increasingly suggests that distributed intelligence—the wisdom of the crowd—is a real thing. A 2013 study in Science, for example, found that golden shiners, a type of schooling fish, could detect gradients as a collective even as individual fish had no sense of the slope. A 2019 paper found that the same fish seemed to sense threats as a group, with the school growing jittery in the presence of a predator. The authors concluded that the school reorganized itself through the structure of its network; the individual fish weren’t more sensitive to danger, but the system was.
“Humans tend to overly focus on the individual,” said Iain Couzin, the director of the Max Planck Institute of Animal Behavior in Konstanz, Germany, and arguably the world’s leading expert on collective behavior. “Even when we think about, you know, intelligence, we focus in on Einstein, we focus in on geniuses. We have this obsession with Steve Jobs as a genius, Einstein as a genius. . . . But in the vast majority of cases, the decisions we make are collective decisions, and the quality of those decisions is not correlated with individual IQ. It’s more about the network of communication.”
And while no one today attributes synchronicity to telepathy, collective behaviors do suggest that there’s more to animal cognition than most of us acknowledge. Researchers have speculated that the incredible speeds at which birds pass information to one another within a flock could mean that individuals aren’t merely responding to the positions and velocities of their closest neighbors, but instead anticipating what those neighbors may do in the future. It’s a feat of mental time travel that’s often considered the exclusive realm of humans.
The strength of Peleg’s and Sarfati’s work, Couzin said, is what it can teach us about the highly specific lives of individual species, as well as synchronicity as a model system. Its results may lead to the recognition of similar patterns in disparate biological systems—and to a fuller understanding of natural selection. “If one zooms out a little bit from fireflies and just thinks more broadly—think about our brains,” he said. “Our brains are basically cells that are firing and influencing others around them that are firing. They’re not flashing in terms of light, but they’re flashing in terms of electricity and chemical pulses, and this creates these patterns and properties at higher levels.”
Couzin, who writes about collective biological systems and the brain, has noted that certain ant colonies exhibit distinctive cycles of rest and activity that resemble neural wave patterns. He’s also curious about whether criticality—which posits that systems can be perched at a point between order and disorder that yields remarkable properties—can explain rapid behavioral changes in flocks and schools. The concept has been integral to statistical physics for more than a century, but has recently been taken up by neuroscientists, who wonder whether it can account for various information-processing characteristics of the human brain. If criticality is behind the high-speed propagation of information across a flock, then it would be evidence of lossless information systems rooted in nature rather than in Silicon Valley.
Steven Strogatz, a professor of applied mathematics at Cornell and the author of Sync: The Emerging Science of Spontaneous Order, believes that the world’s outstanding scientific mysteries, regardless of discipline, are all questions of collectives. Consider how tens of thousands of genes in a cell can interact to cause cancer. Or the fact that the human body has only about thirty thousand genes, yet can produce antibodies in response to an apparently limitless number of foreign substances. Even bacteria organize themselves to produce outcomes that extend beyond that of individual units. Antibiotic resistance, a terrifying public health challenge, is facilitated by colonies of bacteria swapping information across a genomic web.
“What happens when you have a group of about one hundred billion brain cells?” Strogatz asked me. “They start to have consciousness, they start to have emotions. An individual cell is not sad. But put enough of them together, and you get astonishing collective things that we call feelings and memories and perception.”
By late June, Peleg and Sarfati had traded the heat and mosquitoes of Congaree for a brick building in Boulder, where tall windows in the stairwells frame spectacular views of the Flatirons. Along with Peleg’s other postdocs and graduate students, Sarfati maintains a lab that includes an outdoor apiary alongside Boulder Creek. There’s a shared office jammed with desks and equation-covered whiteboards, a windowless cube with a fish tank housing a plant communication experiment, and a basement room stocked with equipment for handling honeybees: a floor sticky from sugary feed, four enormous jars of honey on a tall shelf, and a mesh barrier over the air vents to prevent the bees from escaping.
In another, more cramped room, which required visitors to don hooded beekeeper’s jackets and gloves before entering, they keep X-ray equipment and, at times, bees. The bees emitted a quiet hum, belying the fact that there were approximately ten thousand of them. These particular honeybees hang in dense conical swarms and nimbly respond to stressors by flattening their swarm’s shapes to resemble Frisbees. Peleg’s team was x-raying them to understand how they did so. One little cone about three thousand bees strong hung from a wooden panel a few feet from our waists.
“The best way to connect it to the fireflies would be by trying to understand animal communication based on volatile signals in very large groups,” Peleg said. “So with the fireflies, they create these flash patterns, and you can see how that signal is propagating throughout the entire swarm. And here we’re dealing with either chemical communication with the bees, or mechanical communication. So they’re holding hands, in a sense. Depending on how much force they exert on this cone, they’re going to feel it.” Then all three thousand get the message to reshuffle in a way that stabilizes the structure.
As for Sarfati, he’d gathered enough data on two hard drives to keep him busy with statistics for at least a year. The challenge now, he said as we sat on a bench in the dry mountain air, was less about getting good data than it was about asking interesting questions of it. If Peleg’s experiments represented a bottom-up approach to understanding collective animal behavior by investigating what fireflies did when taken out of the swarm, then Sarfati’s approach was top-down: he’d look at the swarm to find out how each individual works within the group.
His videos of fireflies would allow him to analyze three-dimensional reconstructions of firefly trajectories. A firefly’s flash is obviously a signal, but could there be information encoded in their movements as well? The firefly known commonly as the Big Dipper, for instance, seems to communicate by movement as well as frequency, carving a distinctive J shape in the air as it flashes. Sarfati planned to draw from the math of statistical physics and single-particle tracking to describe what the fireflies did on average, then zero in on the noise—the unknown bits—where he might find the individual animal reacting or thinking: evading a predator, for instance, or responding to a female.
In physics, he explained, some objects, like planets, have trajectories that are completely deterministic. On the other end of the spectrum, there’s Brownian motion, which describes the fully random movements of microscopic particles. Sarfati’s “thinking objects” fall somewhere in between, generating noise that’s both confounding and potentially revelatory. Sarfati was fascinated by the idea that the physical laws governing inanimate objects might—with a few adjustments—also apply to animals; he thought the potential commonalities suggested that those principles contained deeper truths.
Of course, he could just as well discover the opposite. Studies into animals might augment—or at the very least complicate—the laws of physics. Albert Einstein himself pondered this possibility in 1949, in a letter in which he discussed a contemporary’s studies of bees. “It is thinkable,” he wrote, “that the investigation of the behaviour of migratory birds and carrier pigeons may some day lead to the understanding of some physical process which is not yet known.”
Perhaps unsurprisingly, the kind of research that Peleg and Sarfati do is often celebrated less for what it tells us about the physical universe or animals, and more for what it might offer humans. Learning how bees and fireflies solve problems could have applications for communications systems, swarm robotics, and engineering, where it might aid in the development of far-out building materials that flex and adapt under strain. Sarfati’s goals remain less selfish, if not less ambitious. “I’d just like to understand what they do,” he said. “Just the fireflies themselves. I’d like to understand the firefly, for now.”