It is the end of June, and I am wandering about my small island in Maine. I’ve been thinking about the materiality of the world. Today, I just want to experience the fleshiness of this island. I run my hands along the bristly branches of a spruce tree. I could identify that prickle blindfolded. My bare feet sink into spongy moss. On the rocks lie mussel shells dropped from on high by crafty gulls seeking to break them apart and liberate the food within. The shells feel smooth and cool even in the sun. This tiny island in Casco Bay is shaped like a finger, half a mile long and a tenth of a mile wide. A high bony ridge runs down its spine, a hundred feet above sea level, and my house lies on the north end of the ridge. To the south, there are five other cottages, cloaked from one another by a dense growth of trees — mostly spruce, but also pine, cedar, and poplar, whose leaves in the wind sound like hands clapping.
As did Thoreau in Concord, I’ve traveled far and wide on this island. I know each cedar and poplar, each clump of beach rose, each patch of blueberry bush and raspberry bramble and the woody stems of the hydrangeas, all the soft mounds of moss, some of which I touch on my ramblings today. The tart scent of raspberries blends with the salty sea air. Early this morning, a fog enveloped the island so completely that I felt as if I were in a spaceship afloat in outer space — white space. But the surreal fog, made of water droplets too tiny to see, eventually evaporated and disappeared. It’s all material, even the magical fog, like the bioluminescence I first saw as a child. It’s all atoms and molecules.
The materiality of the world is a fact, but facts don’t explain the experience. Shining seawater, fog, sunsets, stars. All material. So grand is the material that we find it hard to accept it as merely material, like meeting a man driving a Cadillac who claims he has only one dollar in his pocket. Surely there must be more. “Nature,” wrote Emily Dickinson,
is what we see —
The Hill — the Afternoon —
Squirrel — Eclipse — the Bumble bee —
Nay — Nature is Heaven.
In the last of these lines, the poet leaps from the finite to infinity, to the realm of the Absolutes. It is almost as if nature in her glory wants us to believe in a heaven, something divine and immaterial beyond nature itself. In other words, the natural tempts us to believe in the supernatural. But then again, nature has also given us big brains, allowing us to build microscopes and telescopes and ultimately, for some of us, to conclude that it’s all just atoms and molecules.
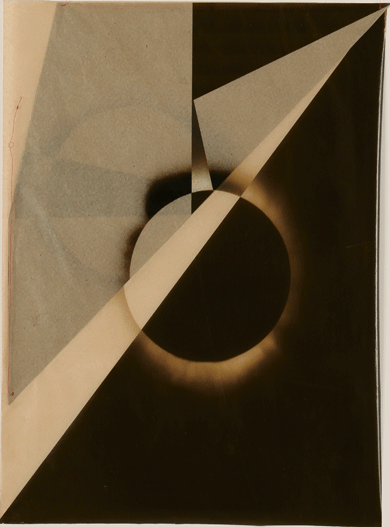
El Sol 2, a collage from the series Koan, by Luis González Palma. For this series, the artist used images of astrophysical phenomena—interplanetary bodies, spectral lines, and stellar particles—from the archives of the observatory at the University of Córdoba, in Argentina. Courtesy Lisa Sette Gallery, Phoenix
For me, the human body is the most amazing and baffling phenomenon of the material world. How could it be that the exquisite and indescribable experience of consciousness, of thought and emotion, of the overpowering sense of an I, is simply the result of so many electrical and chemical flows between neurons, which are themselves nothing but atoms and molecules? I am constantly struck dumb by this mystery. I assume that the first single-celled creatures moving about in the primeval seas did not have consciousness or thoughts. Evidently, those qualities emerged with increasing complexity and natural selection. As Darwin wrote in the last lines of his great book, “From so simple a beginning endless forms most beautiful and most wonderful have been, and are being, evolved.” Beautiful and wondrous, yes — but all material stuff, say the biologists.
It’s a clear summer night and we’ve been sitting on our dock looking up at the stars. Overhead, the diaphanous white sash of the galaxy sweeps across the sky. I feel myself falling into its depths. I am falling and falling, and I am surrounded on all sides by the stars, until I am beyond the Milky Way. In the distance, I see other galaxies, glowing spirals and pinwheels and elliptical blobs, each containing billions of stars. And I myself have grown larger. The galaxies have shrunk to mere dots. I see clusters of galaxies, then clusters of clusters, each appearing for moments and then dwindling away. I am a giant striding through the dark halls of the cosmos, becoming larger and larger, but the universe is always larger still. Mansions within mansions. Space goes on and on and on, and I am dizzy with infinity.
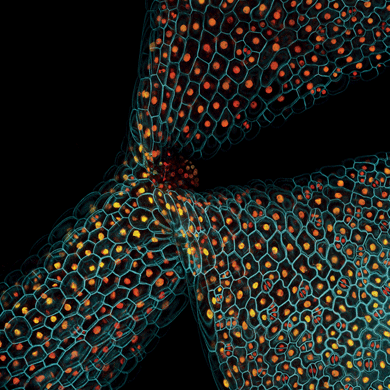
Fluorescent proteins in plants, microscopy by Fernán Federici and Jim Haseloff. Courtesy the artists
Then it reverses. I grow smaller. Dots of light grow into galaxies. I see spirals and pinwheels and elliptical blobs of light, and I am still shrinking. Eventually, I find myself back in my home galaxy, the Milky Way. I can see individual stars, wispy nebulae. I continue to shrink and I hurtle toward a particular star on the outskirts of my galaxy, then toward a particular planet, then toward the dappled brown coast of a landmass on that planet. Finally, I am sitting once again on a wooden dock by the sea. But I continue to shrink. I go inside a leaf, where I see green and blue vessels, veins and ridges, cellular lattices. Conglomerations of molecules. Then I see individual atoms, each a haze of electrical force. Atoms at last, the heralded units of matter for so many centuries.
Is this where my inward-bound journey will end? Have I arrived at the tiniest dots of reality? But there are smaller things still. I fall into a particular atom. I see vibrating mists and vast empty spaces, then a dense throbbing mass down below, at the core of the thing, the protons and neutrons, the nucleus. Relentlessly, I grow smaller. I enter a single proton. It is impossible, the violent energies nearly obstruct my view. Subatomic particles appear out of nothing, like ghosts, then vanish. I see a trio of blurs: the three quarks. Have I finally reached the bottom of existence, the tiniest specks of the world? But there are smaller things still. I shrink within a single quark. I am blinded by energy. Far, far off in the distance, many powers of ten smaller, I see vibrating strings of pure energy. And, astoundingly, I continue to fall. There’s no end to it. I am dizzy with infinity, the infinity of the small.
It may have been the ancient Greeks who first conceived of a tiniest unit of matter, the atom or atomos, meaning “uncuttable.” Atoms, they believed, were not only uncuttable — they were indestructible. Atoms protected us from the whimsy of the gods, said Democritus and Lucretius, because atoms could not be created or destroyed. Even the gods had to obey their iron whims. Newton also prized atoms, but as the handiwork of God rather than as a defense against Him. With a surer grasp of the logic of nature than any mortal before him, Newton wrote: “It seems probable to me that God in the beginning formed matter in solid, massy, hard, impenetrable, moveable particles.” These particles, he speculated, were “so very hard as never to wear or break in pieces; no ordinary power being able to divide what God himself made one in the first creation.” Indeed, atoms were the ultimate Oneness of the material world: perfect in their indivisibility, perfect in their wholeness and indestructibility. Atoms were the embodiment of truth. They were, along with stars, the material icons of the Absolutes.
Atoms also unified the world. A leaf and a human being are made of the same stuff: take them apart, and we find identical atoms of hydrogen and oxygen and carbon and other elements. On that foundation, we can build systems. We can organize and construct the rest of the world. And for a long time, we believed that atoms prevented us from falling forever into smaller and smaller rooms of reality. When we reach atoms — so the thinking went — the falling stops. We are caught. We are safe. And from there, we begin our journey back up, building the rest of the world.
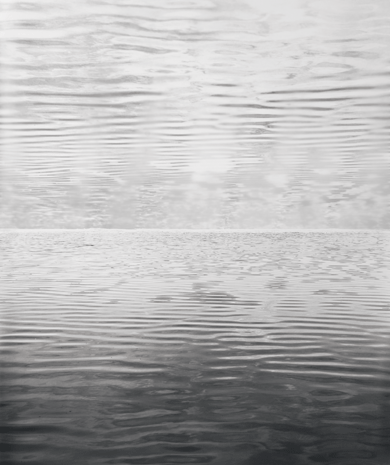
“The Surface of Hopkins Pond Inverted to Print the Sky #8, Mariaville, Maine,” a photograph by Caleb Charland from his series Inversions. Courtesy the artist and Sasha Wolf Projects, New York City
Of course, the idea of fundamental elements can be found in all cultures and eras. Thinkers in ancient India conceived of a system of five: fire, water, wind, space, and earth. Fire was associated with bone and speech, water with blood and urine, earth with flesh and mind. Aristotle, too, built the cosmos out of five elements: earth, air, water, fire, and ether (for the heavenly bodies). For the ancient Chinese, the fundamental elements were wood, fire, metal, water, and earth.
Evidently, we humans are driven to construct the cosmos from such basic elements. Why? Closely related: Why do we create systems and patterns? Are those patterns already there, independent of our desires, or do we impose them on a chaotic universe in order to scratch some existential itch? Could it be that we crave order for sanity? Another thought: with fundamental elements, we can conceive of the world as being constructed, whether the master builder be an active God or the more passive Laws of Nature. A constructed world implies order and design — and the faint suggestion of an intelligence behind that order. Unless that intelligence is in fact our own, looking at ourselves simultaneously through both ends of the telescope.
At an engaging website hosted by the American Institute of Physics, you can listen to the voice of Joseph John Thomson talking about his discovery of electrons in 1897. Electrons were the first attack on the atom. At the time of the recording, in 1934, Thomson was seventy-eight years old and had been for many years the Cavendish Professor of Experimental Physics at Cambridge University. The recording crackles with static, but the words are unmistakable: “Could anything at first sight seem more impractical than a body which is so small that its mass is an insignificant fraction of the mass of an atom of hydrogen?” Impractical indeed! But practicality is beside the point here. We’re talking about a revolution of ideas, a bombing of the palace of Unity and Indivisibility. A photograph of Thomson at the time shows a deadly serious gentleman — balding, with spectacles, a thick walrus mustache, tightly clasped hands, and a starched white collar — staring unflinchingly into the camera as if he were looking two thousand years of history in the eye without apologies. “It was coming sooner or later,” his gaze seems to say. “So buck up and take it like an adult.”
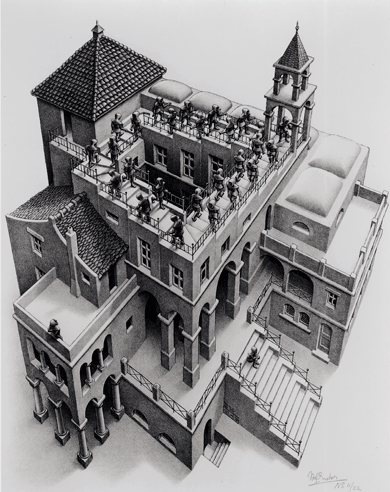
Ascending and Descending, by M. C. Escher © 2018 The M. C. Escher Company, the Netherlands. All rights reserved. www.mcescher.com
Thomson made his discovery by measuring the paths of electrically charged particles as they were deflected by electric and magnetic forces. First, he and others had to develop good vacuum pumps for removing the air in the glass tubes through which the particles moved. Molecules of air would have interfered with the delicate trajectories of the tiny particles under study.
I have a great deal of respect for vacuum pumps. I used them myself during my short-lived encounter with experimental physics as a university student. A vacuum pump, when working properly, starts out with a coarse, grating sound like the chug of a locomotive, graduates to a clicking whine that rises in pitch, and ends with a smooth hum when a good vacuum has been attained. (When the pump is unable to produce a vacuum, it never gets past the chugging locomotive stage.)
The amount of deflection of a charged particle in a good vacuum indicates the ratio of its electrical charge to its mass. From previous experiments, Thomson and others already knew that particular ratio for hydrogen, the lightest of all atoms. What Thomson found was that these other particles, the electrons — which he called corpuscles, and which could be created by heating a piece of metal — had a ratio roughly 1,800 times larger than that of hydrogen atoms. Assuming the same electrical charge, the mass was inferred to be 1,800 times smaller. Evidently, the atom was by no means the smallest unit of matter.
While Thomson was discovering the electron in England, Antoine Henri Becquerel and Marie Curie were discovering the disintegration of atoms in France — what Curie called radioactivity. Becquerel believed that the mysterious radiations recently observed to emanate from uranium, the so-called X-rays, were the result of the absorption of sunlight. The uranium X-rays, in turn, could be detected by nearby photographic plates. Becquerel decided to capture the entire process on February 26, 1896, and prepared his plates accordingly.
On that day, however, Paris was cloudy. Becquerel’s uranium did not receive what he viewed as the required energy of sunlight. On a whim, he decided to develop his photographic plates anyway. To his surprise, they were strongly exposed, showing that the uranium emitted some kind of radiation on its own, without needing to be powered by the sun. Later experiments by Becquerel demonstrated that the X-rays were electrically charged particles of some kind. Curie, meanwhile, found that uranium atoms were essentially hurling out tiny pieces of themselves — and a year later, she detected the same sort of atomic disintegration in another element, radium. Once again, it was clear that the indivisible atom was, after all, divisible. And what lay inside? No one knew. The bottom of the universe had fallen out.
In 1903, the historian Henry Adams mulled over these disturbing developments. “Man’s mind,” he wrote, “had behaved like a young pearl oyster, secreting its universe to suit its conditions until it had built up a shell of nacre that embodied all its notions of the perfect.” But as the twentieth century dawned, he added, “science raised its head and denied”:
The man of science must have been sleepy indeed who did not jump from his chair like a scared dog when, in 1898, Mme. Curie threw on his desk the metaphysical bomb she called radium. There remained no hole to hide in. Even metaphysics swept back over science with the green water of the deep-sea ocean and no one could longer hope to bar out the unknowable, for the unknowable was known.
With his new corpuscles in hand, Thomson proposed what came to be called the “plum pudding” model of the atom: a tiny ball filled uniformly with a pudding of positive electrical charge, into which were sprinkled the negatively charged electrons. You needed the positively charged pudding to balance out the negatively charged electrons, since it was known that most atoms are electrically neutral.
Less than a decade later, a team led by Ernest Rutherford, the great physicist from New Zealand, found that the atom was not a pudding at all. It was more like a peach. A hard pit resided at its center, containing all the positive charge and nearly all the mass. The new particles residing within that hard central pit were called protons and neutrons. Protons have positive electrical charge, neutrons have no charge.
This peach picture emerged after Rutherford’s group fired subatomic particles at a thin sheet of atoms. Some of the particles veered off at large angles, as if they had hit something solid: a hard pit in the atom. With pudding, on the other hand, the deflections should have been small. “It was quite the most incredible event that has ever happened to me in my life,” boomed Rutherford. “It was almost as incredible as if you fired a fifteen-inch shell at a piece of tissue paper, and it came back and hit you.”
The pit at the center of each atom, the nucleus, is not only hard but minuscule: a hundred thousand times smaller than the atom as a whole. To use an analogy, if an atom were the size of Fenway Park, the home stadium of the Red Sox in Boston, its dense central nucleus would be the size of a mustard seed, with the electrons gracefully orbiting in the outer bleachers. In fact, almost the entire volume of an atom, considerably more than 99 percent, is empty space, except for the haze of nearly weightless electrons. Since we and everything else are made of atoms, we are mostly empty space. That vast emptiness is perhaps the most unsettling consequence of dividing the indivisible.
Are we falling and falling without end? Are there unlimited infinities on all sides of us, both bigger and smaller? It is an unpleasant sensation. I am reminded of the M. C. Escher drawing Ascending and Descending, which depicts a line of cloaked men walking around a quadrangle in a medieval castle. The disturbing feature of the picture, achieved through a trick of perspective, is that the walkers are always ascending, marching up a continuously rising staircase, and yet after completing the loop, they end up exactly where they began. It is a staircase without beginning or end. It is a staircase that goes nowhere.
Escher made Ascending and Descending in 1960, at a time when physicists had recently discovered hundreds of novel subatomic particles in the new atom smashers and in high-energy radiations from space. The field of research into elementary particles and forces was thrown into chaos. In addition to electrons and protons and neutrons, there were now delta particles and lambda particles, sigmas and xis, omegas, pions, kaons, rhos, and more. When the Greek alphabet was exhausted, the confounded physicists resorted to using Latin letters. Before, even with the sacred atom fractured, there had been some kind of order. There had been only the electrons and protons and neutrons. But now this howling zoo. There seemed to be no fundamental particles, no bottom to the infinite spiral down, no organizing principles.
Then quarks were discovered in the late Sixties. Temporarily, the plummeting stopped. Each of the hundreds of new particles could be understood as a particular combination of a half dozen basic quarks. Here was a new system for organizing the subatomic zoo! Quarks were the new protons and neutrons — which, in turn, had been the new atoms. I once asked the physicist Jerry Friedman, the codiscoverer of quarks, whether he thought that these particles were the end of the line, the smallest unit of matter. Probably, he answered. He gave reasons. But he hesitated. “I could be surprised,” he said with a grin. “There are always surprises in science.” Surprises in science are always welcome, but sometimes disturbing.
The philosophers of ancient Greece developed a terrifying metaphysical principle called Zeno’s paradox. Suppose you want to walk 15 feet across a room. Before you travel that distance of 15 feet, however, you must go halfway, which is 7.5 feet. And before you go those 7.5 feet, you must travel half that distance, 3.75 feet. And before you go those 3.75 feet . . .
And so on. In their minds, the philosophers kept chopping space into halves: into smaller and smaller dimensions ad infinitum. The indivisible was pitted against the divisible. The ultimate conclusion of this intellectual exercise is that you cannot cross the room. Indeed, you cannot move even an inch. You are frozen in a mental conundrum. You are trapped by the infinity of the small.
When scientists and mathematicians talk about infinity, they are usually imagining a sequence of bigger and bigger spaces and numbers. But infinity, as we have learned, can go in the other direction as well. Tolstoy commented on the problem in an appendix to his book My Religion:
The explanation of everything is sought in those beings which are contained in the microscopic beings, and in those that are in them, and so forth, ad infinitum. . . . The mystery will be revealed when the whole infinity of the small shall be fully investigated, that is, never.
Friedman, a physicist rather than a novelist, is more hopeful. He thinks that quarks may be the end of the line. But there are things we don’t know about quarks. Our current theory of quarks and electrons, called the Standard Model, is known to be incomplete. It does not include gravity. We do have a quite respectable theory of gravity, called General Relativity, but it has not been successfully wedded to the Standard Model. To perform those nuptials, we must develop a theory of gravity that includes quantum physics — so-called quantum gravity. So far, all attempts have failed. But we have ideas about what such a theory would decree about the world within the quark.
The infinitesimal is the key here. Normally, quantum effects are significant only on very small scales, at the size of atoms and smaller, and gravitational effects are important only on large scales, at the size of planets and larger. But it turns out that on really small scales, both quantum and gravitational effects hold sway. This ultra-tiny scale is called the Planck length, named after Max Planck, a pioneer in quantum physics. The Planck length is 10–33 centimeters, a hundred billion billion times smaller than a quark, which is itself a few hundred thousand times smaller than an atom. Another way to visualize the size we are talking about: the Planck length is smaller than an atom by about the same ratio as an atom is smaller than the sun. It is staggering that we have anything at all to say about such impossibly tiny elements of existence.
What are the implications of quantum gravity for the infinity of the small? A critical aspect of quantum physics is that energy and other physical qualities do not come in continuous forms, like a flow of water from the tap, but in discrete units, like raindrops. The quantum raindrops are very, very tiny, so that we are not aware in our world that the flow is actually broken up into tiny parts. Some proposed theories of quantum gravity say that at the Planck scale, space is not continuous but exists in the form of indivisible cells — what one might call Planck cells. Picture a tiny cube, one Planck length on each side. At the Planck scale, space is grainy. And within a Planck cell, space simply does not exist. What we experience as space is the correlation between different corners of these cells.
Planck cells, in other words, would be the atoms of space. Rather than atoms as the smallest unit of matter existing in space, we are now talking about the smallest units of space itself.
Surprisingly, some recent experiments have been able to probe nature at the ultra-tiny Planck length, or so the scientists claim. The graininess of space at Planck should randomly slow down highly energetic light rays, affording a kind of friction as the rays jump from the corners of one Planck cell to the next. (Less energetic rays, with much longer wavelengths, would be oblivious to the Planck cells and move through space as if it were continuous, traveling at the ordinary speed of light.) Researchers using NASA’s Fermi Gamma-ray Space Telescope recently reported a negative result. That is, there was no slowdown of highly energetic light rays originating from a particular astrophysical explosion compared with less energetic light rays coming from the same source. The scientists concluded that if space were indeed grainy, the cell size would have to be much smaller than Planck.
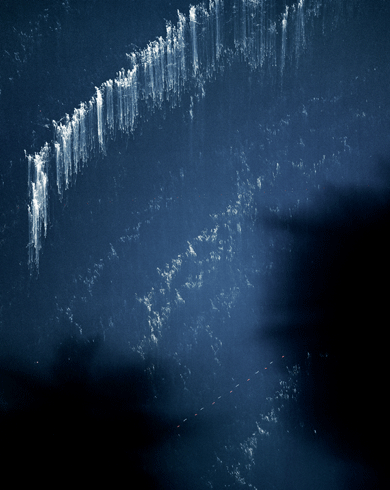
“Camera Placed on My Chest While Laying on the Ground at Night for More Than Two Hours,” a photograph by Caleb Charland from his series Solar Plexus. Courtesy the artist and Sasha Wolf Projects, New York City
Regardless of whether space is indeed grainy at very small scales, physicists are confident that time and space must be chaotic at Planck. Because of the indeterminate, probabilistic character of quantum physics, at the dimensions of the Planck length, space and time churn and seethe, with the distance between any two points wildly fluctuating from moment to moment. Indeed, at the Planck scale, time itself randomly speeds up and slows down, perhaps even going backward as well as forward. In such a situation, time and space no longer exist in a way that has meaning to us. The sensation of smoothness and substantiality that we experience in our large world of houses and trees results only from averaging out this extreme lumpiness and chaos at the Planck length, in the same way that the graininess of a beach disappears when seen from a thousand feet up.
Thus, if we relentlessly divide space into smaller and smaller pieces, as did Zeno, searching for the smallest element of reality, we arrive at the phantasmagoric world of Planck — where space no longer has meaning. Instead of answering the question of what is the smallest unit of matter, we have invalidated the words used to ask the question. Perhaps that is the way of all ultimate reality, if such a thing exists. As we get closer, we lose the vocabulary. Sitting at midnight on my wooden dock by the sea and imagining myself falling and falling into smaller rooms of reality, I might continue to fall without limit. But once I reach Planck, space as I know it no longer exists. Space has been blown thin by an ancient glassblower, so thin that it dissolves into nothingness. The Planck world is a ghost world. Perhaps that is where we must look for the Absolutes, even if we no longer have the words to describe them.